The world’s first heavy-ion colliding beam machine, the Relativistic Heavy Ion Collider (RHIC) at Brookhaven, came on line late last year. At a recent conference, RHIC experiments revealed the initial results of exploring nuclear behaviour in this new energy regime.
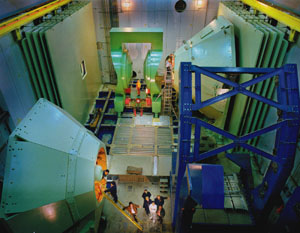
Following the commissioning last year of Brookhaven’s Relativistic Heavy Ion Collider (RHIC), the Quark Matter 2001 conference, held on 15-20 January at the State University of New York (SUNY), Stony Brook, and organized jointly by Brookhaven and Stony Brook, provided the first shop window for results under these new physics conditions.
RHIC is the world’s first heavy-ion colliding beam machine. In these colliders, the figure of merit is the collision energy per nucleon pair (E/A, where A is the atomic number of the nucleus), and RHIC reaches higher E/A than had previously been possible. The results presented at QM 2001 came from just one month of RHIC running in late 2000 with gold nuclei (A = 197), at E/A = 130 GeV. The machine luminosity, a measure of the collision rate, was 0.2 x 1026/cm2/s, which is one-tenth of RHIC’s design figure.
Also presented at the meeting were the latest results from heavy-ion experiments at other machines, notably from CERN’s fixed target programme at the SPS synchrotron, where E/A ranges from 9 to 17 GeV with nuclei up to lead (A = 208) were recorded, and from Brookhaven’s Alternating Gradient Synchrotron, where E/A ranged from 2 to 6 GeV with gold nuclei.
Rising to the central plateau
Nucleus-nucleus and proton-proton collisions should be compared at the same value of E/A. To a first approximation, the overall total particle production (multiplicity) in a nucleus-nucleus collision should resemble A times that of a proton-proton collision. Thus an understanding of nucleus-nucleus collisions in the RHIC requires a similar understanding of comparable behaviour in proton-proton collisions.
In the 1970s, proton-proton collision at collision energies of 20-60 GeV were studied at CERN’s Intersecting Storage Rings, which found a marked change at around 20 GeV.
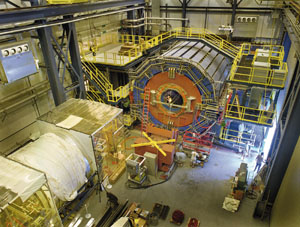
If the produced particle multiplicity is measured as a function of rapidity, y (a measure of production angle), the multiplicity is symmetric about y = 0 (perpendicular to the colliding beam axis). At 17 GeV, there are about two produced hadrons per unit rapidity at y = 0, where the multiplicity is greatest, and an emerging central plateau, out to y = ±1, with the multiplicity falling to zero by about y = ±2.5. For proton-proton collisions, by 130 GeV there are about three produced hadrons per unit y with a central plateau, this time extending to y = ± 2.
Seeking the central plateau
In nucleus-nucleus collisions under CERN SPS conditions, no such central plateau had yet emerged, the total multiplicity being a single peak around y = 0, falling quickly on either side. At y = 0 there are about 500 produced hadrons per unit y, approximately 50% higher than would be expected from simply A times the proton-proton behaviour.
At RHIC, however, the central plateau becomes evident in nucleus-nucleus collisions. The PHOBOS detector finds that this extends over y = ± 2, in a total distribution going out to y = ± 5. There are some 900 (± 10%) hadrons per unit y at y = 0, again about 50% higher than simply A times the proton-proton behaviour. Theoretical estimates of the produced particle multiplicity at RHIC were generally higher than those found experimentally, although some models (e.g. EKRT and HIJING) were close.
Once the central plateau opens up, the y = 0 behaviour changes dramatically. The fraction of net baryons plummets from 14% at SPS conditions to just 3% at RHIC. The ratio of antiprotons to protons jumps from 0.1 at the SPS to 0.65. This latter figure was found by all four RHIC detectors – BRAHMS, PHENIX, PHOBOS and STAR – and is comparable to the behaviour seen in proton-antiproton collisions.
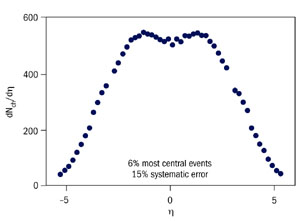
Can the central plateau be used as a laboratory to measure the behaviour of constituent quarks and gluons? The radius of a nucleus of atomic number A is A1/3 that of a proton, so in high-energy nucleus-nucleus collisions one can study proton-proton collisions in a transverse volume up to A2/3 larger. As A increases (very large nuclei), so does the transverse volume, and perhaps central nucleus-nucleus collisions reach equilibrium at a certain temperature.
Simulations of quark-gluon field theory (quantum chromodynamics) using a hypothetical lattice (see Workshop looks through the lattice) show a sudden rise in “pressure” at a critical “temperature” of 175 MeV. The increase above this value is because quark and gluon constituents have many more degrees of freedom than composite particles like pions.
Studying new effects
Are this and other lattice predictions seen in nucleus-nucleus collisions? Even from the thin sliver of RHIC data presented at QM2001, it is clear that there are pronounced differences in the spectrum of produced particles in nucleus-nucleus, compared with proton-proton, collisions.
A change in the spectrum of particle production with transverse momentum is very evident. Above 1.5 GeV, the number of neutral pions found by PHENIX decreases sharply with increasing transverse momentum. STAR and PHENIX find a similar but less dramatic suppression for all charged hadrons. This suppression is always at least 50% of what is expected from A times the proton-proton behaviour.
Explaining this and other gross features is a challenge. At soft momenta, nucleus-nucleus collision behaviour at both RHIC and the SPS differs from the proton-proton case. In this region, particle spectra can be fitted to a thermal distribution with a final temperature related to pion “freezeout” and a relative velocity of the thermal bath. Below about 0.5 GeV transverse momentum, the STAR detector finds that the final temperatures at the SPS and RHIC are about the same – around 100 MeV. The velocity, however, increases sharply.

Elliptic flow, related to asymmetries in peripheral collisions, increases markedly between the SPS and RHIC energies, the corresponding asymmetry parameter increasing from 3.5% to 6%.
Looking for new horizons
Another surprise from January’s Quark Matter conference was that system sizes, as measured by particle interferometry, do not change drastically. Classic Hanbury Brown-Twiss interferometry gives an estimate of the size of the system that last emitted two identical particles, such as neutral pions. Radii increase by no more than 10-20% (to about 6 fm) from SPS to RHIC conditions (PHENIX and STAR). However, the “lifetime” of the system is brief and, while some models had predicted that this would increase, at RHIC, big nuclei appear to blast apart just about as fast as they can.
Another criterion of interest is the fluctuation in behaviour on an event-by-event basis. Some signs of this had been seen at the SPS, but at RHIC this appears to increase dramatically.
At QM 2001, new results also appeared from continuing analysis of the SPS experiments’ data, where the anomalous suppression of J/psi particles seen by the NA50 experiment and the change in shape of the dilepton spectrum below the rho peak, seen by the CERES experiment, are notable (see Heavy implications for the first
second).
For 2001, RHIC is scheduled to run at its full collision energy of 200 GeV per nucleon with gold beams at the machine’s design luminosity. Polarized (spin-oriented) proton-proton collisions should also be on the menu. Given the interesting initial results, it is important also that RHIC should increase its energy coverage stepwise, and in the type of beam used, for only in this way can changes in behaviour, such as those already noted, be tracked and explained.