A project to test proton-driven plasma wakefield acceleration.
Résumé
AWAKE : des hautes énergies en un clin d’œil
L’accélération par champ de sillage plasma entraînée par des protons pourrait permettre l’accélération d’électrons à l’échelle du téra en une seule cellule plasma. C’est pour mettre à l’épreuve cette approche novatrice qu’a été lancée au CERN l’expérience de démonstration du principe AWAKE (accélération par champ de sillage plasma entraînée par des protons). Le projet utilisera des paquets de protons de 400 GeV issus du Supersynchroton à protons ; ce sera la première expérience d’accélération par champ de sillage entraînée par un faisceau en Europe, et la première expérience de ce type s’appuyant sur un faisceau de protons au monde. Les résultats auront un impact considérable sur les futures expériences de R&D utilisant cette technique, qui seront utilisées à plus grande échelle et qui pourraient consister par exemple en l’accélération de paquets d’électrons à environ 100 GeV sur une centaine de mètres.

To complement the results that will come from the LHC at CERN, the particle-physics community is looking for options for future lepton colliders at the tera-electron-volt energy scale. These will need to be huge circular or linear colliders. With the accelerating gradients of today’s RF cavities or microwave technology limited to about 100 MV/m, the length of the linear machines would be tens of kilometres. However, plasma can sustain much higher gradients and the idea of harnessing them in plasma wakefield acceleration is gathering momentum. One attractive idea is to use a high-energy proton beam as the driver of a wakefield in a single plasma section.
To verify this novel approach, a proof-of-principle R&D experiment – the Advanced Wakefield Experiment (AWAKE) – has been launched at CERN, using 400 GeV proton bunches from the Super Proton Synchrotron (SPS). AWAKE will be the first beam-driven wakefield-acceleration experiment in Europe and the first proton-driven plasma-wakefield-acceleration experiment in the world. The results will have a significant impact on future larger-scale R&D on this technique, for example in accelerating electron bunches to around 100 GeV in 100 m.
Previous research on the potential of plasma as a medium for high-gradient acceleration has focused on injecting a short, intense laser pulse or an electron bunch into a plasma cell. With laser excitation, electrons have been accelerated to 1 GeV in 3 cm, with a gradient of 33 GV/m (CERN Courier November 2006 p5). In other experiments using an electron bunch as driver, the energy of particles in the tail of a bunch was doubled from 42 GeV to 85 GeV in 85 cm – corresponding to a gradient of 52 GV/m (CERN Courier April 2007 p5). However, the energy gain in these studies is limited by the energy carried by the laser or electron drive beam (<100 J) and the propagation length of the driver in the plasma (<1 m). Therefore the staging of a large number of acceleration sections would be required to reach the interesting region of 1 TeV per particle or more.
Proton beams of the kind produced at CERN could overcome the issue of staging. In the SPS, beams with 3 × 1011 protons per bunch at 400 GeV/c carry much higher energy (19 kJ). This would drive wakefields over much longer plasma lengths than other methods and consequently proton drivers can take a witness beam to the energy frontier in a single plasma cell. Simulations have shown that an LHC-type proton bunch (1 TeV, 1011 protons) with an rms bunch length of 100 μm can accelerate an incoming 10 GeV electron bunch to more than 500 GeV in around 500 m of plasma with an average gradient ≥1 GV/m (Caldwell et al. 2009, Lotov et al. 2010).

To reach accelerating gradients of a gigavolt per metre or more requires plasma densities where the number of electrons, ne, is of the order of 1015 cm–3. At these intensities the plasma wavelength, λpe, is about 1 mm. To achieve the maximum electric field in the plasma, the drive beam requires short, densely packed proton beams with a longitudinal bunch length, σz, of the same order as λpe.
The proton bunches available today are much longer – with σz around 10 cm – and producing bunches as short as 1 mm is not possible without major investment. However, the effect known as self-modulation instability (SMI) provides fortuitous opening of the path to an immediate experimental investigation of proton-driven wakefield acceleration with the existing proton bunches at CERN. A proton beam propagating in plasma produces micro-bunches that are generated by transverse modulation of the bunch density. These micro-bunches are naturally spaced at λpe and so resonantly excite a strong plasma wave (figure 1). Recent studies have shown that wakefields at high amplitudes – similar to what would be driven if all of the charge were in a single short bunch – can be achieved with a modulated long proton bunch (Kumar et al. 2010, Caldwell et al. 2011).
The AWAKE experiment
The AWAKE experiment at CERN was proposed by an international collaboration that is today made up of 13 institutes – including the Budker Institute of Nuclear Physics, CERN, MPI Munich and University College London – and numbers more than 50 engineers and physicists. In addition, several more institutes have expressed interest in participating in AWAKE. The collaboration first outlined the proposed experiment in a letter of intent that was presented to the SPS Committee in 2011. This was followed in March 2013 by a technical design report that was submitted to the CERN management and to the SPS Committee (Caldwell et al. 2013). A positive review and recommendation of the project led to approval of the AWAKE experiment by the CERN Research Board in August.
The measurement programme of the AWAKE project includes benchmark experiments using proton bunches to drive wakefields and to understand the physics of the proton self-modulation process in the plasma. It will also probe the accelerating wakefields with externally injected electrons, study the injection dynamics and production of multi-giga-electron-volt electron bunches, and develop long, scalable and uniform plasma cells and schemes for the production and acceleration of short proton bunches.
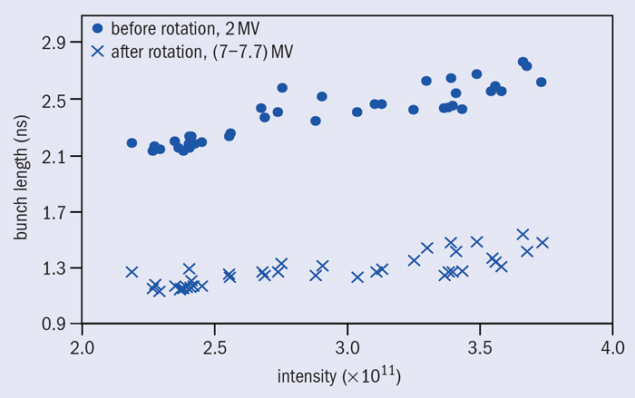
Figure 2 shows the conceptual design of the AWAKE experiment. An LHC-type proton bunch of 400 GeV/c but with higher intensity (around 3 × 1011 protons per bunch) and with a longitudinal rms length, σz, of 9–12 cm will be extracted from the SPS and sent to the experiment, where it will be focused to a transverse dimension of 200 μm near the entrance of a plasma cell. A 2 TW laser pulse co-propagating within the proton bunch creates the plasma by ionizing the (initially neutral) gas in the plasma cell. This sudden plasma creation seeds the SMI of the proton bunch. The SMI develops in the proton beam over the first few metres of the plasma, then saturates and the bunch becomes self-modulated. After the SMI saturation, an electron beam with 109 electrons, energy of around 16 MeV and with σz, electrons >λpe will be side-injected at a small angle (a few milliradians). A fraction of the electrons will be trapped in the wakefield and accelerated.
For the first experiments, the plasma cell will be a 10-m-long rubidium vapour source, with the necessary longitudinal density uniformity of around 0.2%. With the current AWAKE baseline parameters, simulations show that the electrons could be accelerated to energies higher than 2 GeV, with an energy spread of a few per cent and achieving a gradient of 0.1–1.2 GV/m along the 10-m-long plasma.
At a later stage the experiment will use two plasma cells and on-axis electron injection. The first cell will preserve the SMI seeding ability and the second will be used for electron acceleration in the wakefields that are resonantly driven by the modulated proton bunch. Introducing a step in plasma density during the growth of the SMI could maintain the wakefields at the level of gigavolts per metre over distances of several metres.
Short, high-intensity bunches have already been studied at the SPS and the scaling of bunch length and transverse emittance as a function of beam intensity has been identified to guide the design parameters of the AWAKE project (Argyropoulos et al. 2013). To obtain a short longitudinal bunch length, the bunches are rotated in longitudinal phase space using the maximum available RF voltage before extraction. Figure 3 shows the bunch length measured before rotation, at 2 MV, and after rotation, at 7–7.7 MV.
The AWAKE experiment will be installed in the existing CERN Neutrinos to Gran Sasso (CNGS) facility (CERN Courier November 2006 p20). This deep underground area, which is designed for running an experiment with a proton beam of high energy and intensity, has a 750-m-long proton beamline that is optimized for a fast extracted beam from the SPS at 400 GeV/c. Figure 4 shows the integration of the AWAKE experiment in the experimental area, with the plasma cell installed in the downstream end of the CNGS proton-beam tunnel and upstream of the CNGS target.
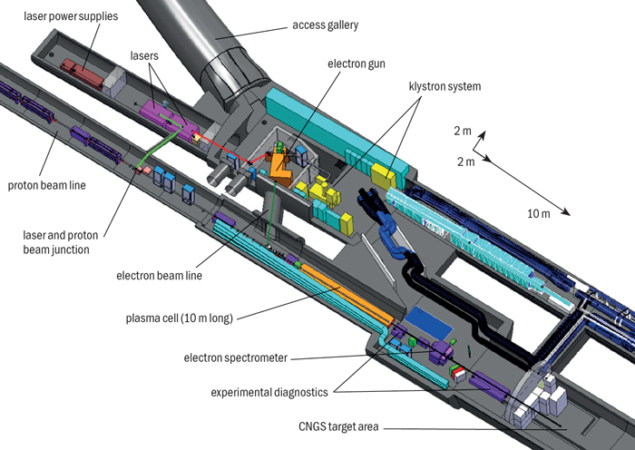
Although the facility already exists, there are several issues that need to be tackled to set up the AWAKE experiment in this area. Essential modifications to the end of the proton beamline include changes to the beam instrumentation and to the final focusing system, in addition to integration of the laser and electron beam with the proton beam. General services such as cooling and ventilation, electricity, radiation monitoring and an access system exist and are operational but some changes will be necessary to adapt them to the AWAKE set-up.
The laser system will be housed in an area that is modified to be dust free and temperature regulated. The high-power laser beam will be transported through a new dedicated tunnel connecting the laser area to the proton-beam tunnel. The area adjacent to the experimental area will be modified to house the electron source and its klystron powering system. The electrons will be transported from the source to the proton-beam tunnel along a beamline through a new 7-m-long liaison tunnel before being injected into the plasma cell. This electron beamline is designed for both side injection (long electron bunches) and on-axis injection (short electron bunches).
The electron source will be driven by a laser pulse that is derived from the same laser system used for the plasma ionization, which will require a synchronization between the laser pulse and the electron beam at a level below 1 ps. The desired synchronization of around 100 ps between the proton beam and the laser beam will be achieved by re-phasing and locking the SPS RF to a stable mode-locker frequency reference from the laser system.
To measure the properties of the electron acceleration, a state-of-the-art magnetic spectrometer with large momentum acceptance (10–5000 MeV) and good momentum resolution will be installed downstream of the plasma cell. A scintillating screen connected to a CCD camera will show the electrons exiting the spectrometer. For the proton beam, various diagnostics will measure the self-modulation effects downstream of the plasma cell. The protons will then be dumped in the existing CNGS beam dump, which is 1100 m downstream of the experimental area, therefore avoiding any radiation into the AWAKE region.
With official approval of the AWAKE experiment in August, the collaboration is now fully “awake” and the first protons can be sent to the plasma cell at the end of 2016. This will be followed by an initial three-to-four-year experimental programme with four periods of data-taking annually, each lasting two weeks.