This summer DESY celebrated the discovery in 1979 of the first direct evidence for gluons in experiments at the electron-positron collider, PETRA. Talks at a special symposium provided some personal views of 25 years of quarks, gluons and quantum chromodynamics.
Twenty-five years ago experiments at DESY provided the first evidence for a very special kind of particle collision: an electron- positron annihilation process with three “coplanar jets”, i.e. three collimated bundles of particles heading away in the same plane from the electron-positron collision point, rather like the prongs of the Mercedes symbol. Using the PETRA storage ring, which had been completed only the previous year, the teams working at PETRA had found the first direct experimental proof of the existence of the gluon – the particle that transmits the strong force. Analysis of the three-jet events showed that two of the three particle jets were produced by a quark-antiquark pair; the third was generated by a gluon.

This summer DESY commemorated the discovery with a special colloquium, “Gluons and Quantum Chromodynamics”, which was held in the laboratory’s main auditorium on 7 June. In his opening address to the 400 guests, Hermann Schunck, the representative of the German Federal Ministry of Education and Research, emphasized the consequence of the finding. “The discovery of the gluon at PETRA here at DESY marks a truly epochal point in the history of physics – mirroring the pivotal role of the gluon in the realm of physics comparable to the other exchange particles, the photon and the W and Z bosons,” he said. He then left the floor to the three scientific speakers of the colloquium – Harald Fritzsch from Munich, Gerard ‘t Hooft from Utrecht and Albrecht Wagner, the director-general of DESY. In turn, they recalled the emergence of the ideas of quarks, gluons and quantum chromodynamics (QCD), described the structure of QCD theory and reviewed the ground-breaking experiments that led from the discovery of the gluon to the establishment of QCD as the accepted theory of the strong interaction.
The emergence of QCD
As Fritzsch emphasized, QCD is a most exceptional theory in that it generates an enormous complexity out of a very simple Lagrangian – describing for instance all atomic nuclei out of essentially one parameter. It is well established today and has moved on from the era of testing into the realm of precision physics. The route, however, has been long and often paved with misunderstandings and false starts. After the success of quantum electrodynamics in describing the electromagnetic interaction in the 1940s, the general mood turned to one of confusion.

Particle after particle appeared in experiments and the particle-physics garden, which had seemed so tidy in the 1940s, grew into a jungle during the 1960s. A major breakthrough came in 1964, when Murray Gell-Mann and George Zweig proposed that all hadrons are in fact composed of even more elementary constituents, which Gell-Mann called quarks. At the end of the 1960s deep-inelastic scattering experiments at the Stanford Linear Accelerator Center (SLAC) showed for the first time that these quarks were not just hypothetical mathematical entities, but indeed the true building blocks of hadrons.
There was a theoretical objection to the quark model, however; it appeared to violate the Pauli exclusion principle, which states that no two particles with half-integer spin can occupy the same state. Thus the Δ++ particle, which was supposed to consist of three identical quarks in the same state, seemed to be inconsistent with the Pauli principle. A solution to the problem was proposed in 1964 by Oscar Greenberg, and later elaborated in its final form by Fritzsch, Gell-Mann and Heinrich Leutwyler. They suggested that quarks actually come in three “colours”, and that the only stable states formed by them are colourless combinations – a hypothesis that sounded at first like sleight of hand, but which in fact proved to be a most fruitful idea, providing the basis for what later became known as QCD, the mathematical description of the strong interaction.
The quark model, and with it the QCD gauge theory of the strong interaction, gained further impetus in the 1970s, as ‘t Hooft described at the colloquium. In seminal work that revolutionized the theoretical background of particle physics and earned him the 1999 Nobel Prize in Physics together with Martinus Veltman, ‘t Hooft showed in 1971 that such “non-abelian” gauge theories are renormalizable. He thus eliminated a fundamental problem that had hampered the development of a mathematical description of the strong interaction for years, and paved the way for the development of the complete gauge theory of QCD by Fritzsch and Gell-Mann in 1972. Two years later the discovery of the J/ψ meson marked what became known as the November revolution – the discovery of a fourth type of quark, and as such an eminent confirmation of the quark model and QCD.
The discovery of the gluon
In the late 1960s and early 1970s experiments had thus provided evidence for the reality of the quarks. The gauge theories describing the various interactions, however, predicted the existence of mediator bosons that transmit the forces between the particles. Of these, only the photon was definitely known at that time. A first hint of gluons, the mediators of the strong force, came from deep-inelastic scattering experiments, which had shown that only half of a proton’s momentum is carried by the quarks. The missing momentum fraction was interpreted as being carried by electrically neutral constituents, presumably the gluons. But how could the actual existence of these gluons be demonstrated experimentally?
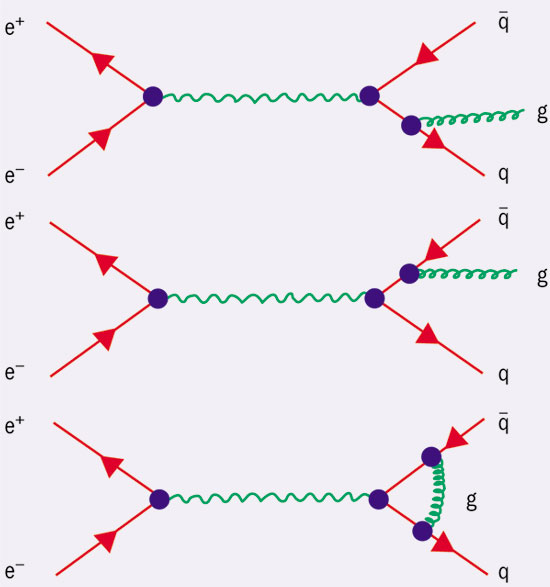
As Wagner – himself an experimenter on the JADE experiment at PETRA at the time – recollected in his talk, by the end of the 1970s it was widely accepted that the annihilation of an electron and a positron and the subsequent formation of a quark-antiquark pair proceeded primarily via the exchange of a photon. The generated quark-antiquark pair would then fragment into hadrons, which would appear in the detector as two back-to-back hadron jets with limited transverse momentum and increasing momenta along the jet axis. Such events with two hadron jets had been discovered at the SPEAR storage ring at SLAC in 1975, and were later analysed in detail at DESY’s 5GeV storage ring, DORIS. Interestingly, just before PETRA appeared on the scene, the PLUTO experiment at DORIS, running on the Y(1S) b-bbar resonance, showed event topologies that were distinctly different from those generated in the nearby continuum, suggestive of the conjectured three-gluon decay of the 1S b-bbar state.
The idea of searching for gluon jets had actually been proposed by John Ellis, Mary Gaillard and Graham Ross in a seminal paper that appeared in 1976. Under the apparently imperative title “Search for Gluons in e+-e– Annihilation”, the authors suggested the existence of “hard-gluon bremsstrahlung”, which should give rise to events with three jets in the final state. According to the laws of field theory, the outgoing quarks can radiate field quanta of the strong interaction, i.e. gluons, which should in turn fragment into hadrons and thus create a third hadron jet forming a plane with the other two (see figure 1). At the particle energies of up to 15GeV per beam delivered by DESY’s newly built PETRA electron-positron storage ring, the probability for such hard-gluon bremsstrahlung processes to occur might amount to a few percent.
The PETRA machine had been completed by the summer of 1978 after only two years of construction. While still being commissioned, it had delivered the first events for e+e– hadrons to the detectors at the end of 1978. Six months later, at the International Neutrino Conference in Bergen, Norway, on 18 June 1979, Bjørn Wiik reported on an event that had been observed in the TASSO detector at PETRA only a few days earlier. It had been analysed in detail by his colleague Sau Lan Wu and her co-worker Georg Zobernig. While the TASSO team was observing abundant events with the expected two-particle jets created by the outgoing quark-antiquark pair, Wu and Zobernig – who had designed a fast algorithm for the analysis of more complicated event topologies, in particular multi-jet structures – had uncovered something new: an event that clearly involved three jets whose momenta lay in a plane. When Paul Söding, who belonged to the same team, travelled to Geneva two weeks later for the European Physical Society (EPS) conference, he was already able to present a few of these three-jet events (see figure 2,). Moreover, a whole variety of plots displaying various analyses gave convincing evidence that bremsstrahlung of the gluon, which had been postulated as the boson mediating the strong force, had indeed been discovered.
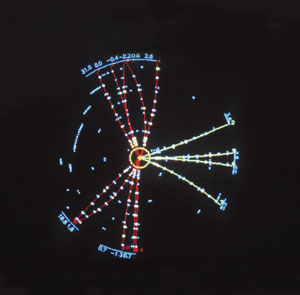
Shortly afterwards similar three-jet event topologies were announced by JADE, MARK J and PLUTO, the other groups working at PETRA. All four collaborations presented their data at the Lepton-Photon Symposium at Fermilab in Chicago in August 1979. The corresponding publications by TASSO, MARK J and PLUTO followed in autumn 1979, while the JADE Collaboration published an extended analysis, which included a first determination of the strong coupling constant αs(q2), in spring 1980 (MARK J Collaboration, PLUTO Collaboration and TASSO Collaboration 1979; JADE Collaboration 1980). Sixteen years later, in July 1995, the discovery of the gluon was honoured by the EPS, which awarded its Prize for High Energy and Particle Physics to four physicists representing the TASSO Collaboration: Paul Söding, Bjørn Wiik, Günter Wolf and Sau Lan Wu. A special complementary prize was also awarded to the four collaborations in recognition of their combined work, since, as the EPS statement reads, the “definite existence (of the gluon) emerged gradually from the results of the TASSO Collaboration and the other experiments working at PETRA, JADE, MARK J and PLUTO”.
As Wagner emphasized, the results obtained by the four experiments and the speed with which they were achieved would have been impossible without the initiative of DESY’s director-general at the time, Herwig Schopper, and the outstanding work of the accelerator physicists and engineers under the leadership of accelerator division director Gustav-Adolf Voss, who managed to complete the accelerator on budget and in record time, six months ahead of schedule.

The discovery of the gluon marked the beginning of intensive tests of QCD at PETRA. These included the determination of the spin of the gluon, which proved to be a vector particle; the so-called string effect, i.e. the hadronization of quarks and gluons via the formation of colour strings; various tests of second-order QCD calculations (see figure 3); and precise determinations of the running strong coupling constant αS. At the end of the 1980s the baton passed on to CERN’s Large Electron Positron (LEP) collider. Although it was primarily built to perform precision tests of the electroweak force through the production and decays of Z and W bosons, due to the very large event sample, LEP became an excellent testing ground for QCD (CERN Courier May 2004). For example, whereas the experiments at PETRA were unable to distinguish between quark-quark-gluon and gluon-gluon-gluon vertices, these differences were measured at LEP’s OPAL experiment using a sample of 4 x 106Z0 decays.
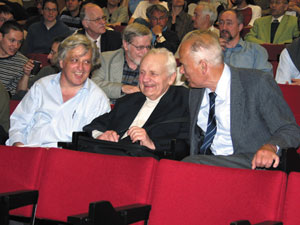
Today, QCD is put through its most stringent tests at the Tevatron proton-antiproton collider at Fermilab and at DESY’s electron-proton collider, HERA. The advances made have been remarkable, ranging from an extremely precise determination of the proton structure function to the study of the origin of nucleon spin, the exploration of the non-perturbative nature of the strong interaction, or the problem of quark confinement. Over the past 25 years QCD has thus emerged as the uniquely successful theory of the strong interaction, and it is as such a full part of the Standard Model of particle physics. As Fritzsch concluded in his talk at the colloquium, “The phenomena of the strong interaction are now ‘in principle’ understood.” With the advent of new theoretical approaches such as QCD lattice calculations and new experiments at future accelerators, the “still impressive list of unsolved QCD problems” is set to shrink fast.
• This article is based on one that is to appear in Europhysics News and is published with permission.