Fine structure in the energy spectrum provides new clues.
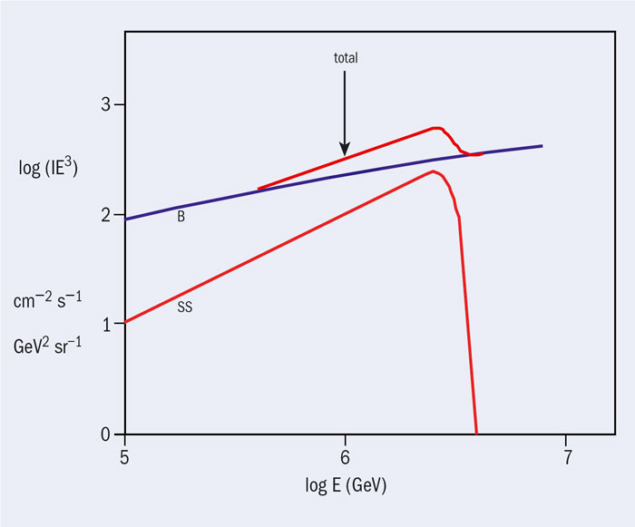
As 2012 approaches, and with it the centenary of Victor Hess’s famous discovery, it really is time that we found out where cosmic rays originate. Gamma-ray astronomy has shown that most of the particles come from the Galaxy, but even this discovery was 63 years in coming (Dodds et al. 1975). Supernova remnants (SNR) have long been suspected to be the source of cosmic rays below about 100 PeV, the production mechanism being Fermi acceleration in shock-borne magnetic fields. The energies involved are reasonable: about 1043 J into cosmic rays per SNR per century. However, there are doubts, with pulsars or extended sources being other possibilities.
The origin problem arises because magnetic fields on a variety of scales in the Galaxy cause particles at these energies to pursue tortuous paths, so that their arrival directions at Earth bear virtually no relationship to the directions of the sources. Solving the problem therefore requires other approaches. Structure in the energy spectrum could provide such a possibility.
Clues from the energy spectrum
The only feature of the cosmic-ray energy spectrum that researchers currently agree on is a steepening that starts in the region of 3–5 PeV. First observed by German Kulikov and George Khristiansen at Moscow University around 50 years ago (Kulikov and Khristiansen 1958), this so-called “knee” has been confirmed time and again from the 1960s onwards. It was not until 13 years ago, however, that we pointed out that the “knee” is too sharp for a conventional explanation in which the galactic magnetic field gradually “loses its grip” on the particles (Erlykin and Wolfendale 1997). We argued instead that it results from a dominant contribution from a single, nearby source. The idea is that particles from the single source provide a component that pokes through the background arising from an amalgam of many differing sources (figure 1).
The single-source model has had a rough ride, with most researchers being unwilling to accept that there could be “fine structure” in the spectrum caused by nuclei heavier than protons from this source. Our early analysis was based on extensive air-shower (EAS) data from a variety of EAS arrays. While these results are still valid, we have recently analysed new data from some 10 arrays, thus extending the reach to higher energies than before. Remarkably, and importantly, a new feature has appeared at about 70 PeV.
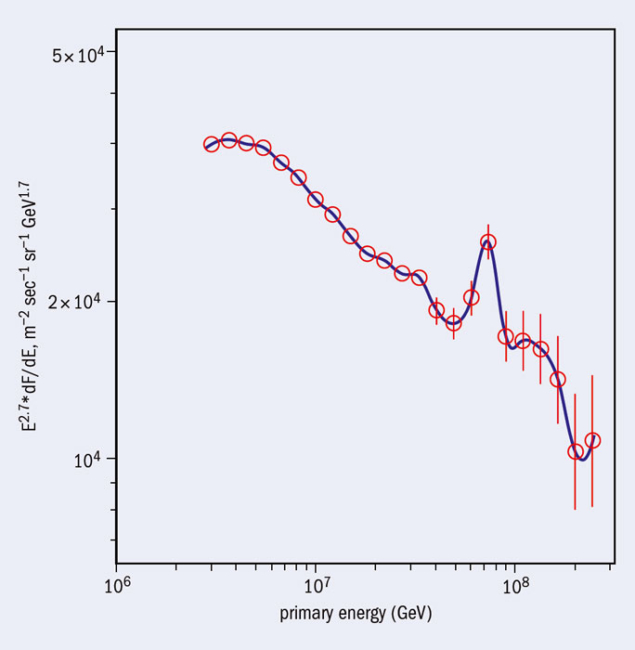
When the energy spectrum is plotted as log(E3I(E)) versus logE, a “knee” will appear as a peak and it is a new peak in the spectrum plotted in this way that is of interest (figure 2). It was first reported by the GAMMA collaboration led by Romen Martirosov, using the GAMMA EAS array of the A I Alikhanyan National Science Laboratory at Mount Aragats in Armenia (Garyaka et al. 2008). Our own survey shows that it is also present in most of the other reported spectra (Erlykin and Wolfendale 2010). It is interesting to note that our first paper on the single-source model showed a small excess at the level of 2.6σ just where the GAMMA collaboration finds its 70 PeV peak. However, we did not claim that this small peak was significant at the time.
We have now used these recent spectra to investigate the case for SNRs in general as the source of cosmic rays at energies below 100 PeV. The model for the acceleration of the cosmic rays predicts that those with charge Z should have a differential energy spectrum, with a negative slope of about 2, up to a maximum energy proportional to Z. Nuclei are conventionally grouped into the following nuclear bands: P, He, CNO, M(Ne, Mg, Si) and Fe (actually Fe and Ni). The spectrum expected at some distance from the single source differs from that at the SNR itself because of propagation effects, but these can be calculated. This is what we have done, assuming that the single source is Monogem, a “recent” SNR with an age of 85–115 ky, at a “local” distance of 250–400 pc (Erlykin and Wolfendale 2003).
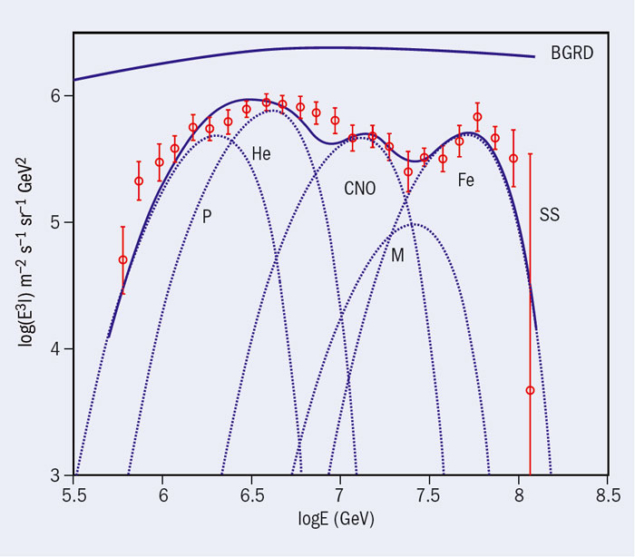
Figure 3 shows a synthesis of the 10 reported spectra from the EAS arrays from which the (predicted) smooth background, also shown, has been subtracted. The resulting “spectrum” is thus our estimate of the extra contribution from the single source. The figure also shows our fits of the individual single-source spectra in the different nuclear bands to the observations. Inevitably, there is no question of a perfect fit: although the He and Fe peaks seem well founded, those for CNO and P are less well so. Peaks for P and He have been seen in other experiments, however. The whole range is thus reasonably well represented.
Our calculations give the relative abundances at a fixed energy per particle of the various nuclear groups on ejection from the single source as: P(0.477), He(0.406), CNO(0.081), M(0.010) and Fe(0.026). Remarkably, with the exception of the M group, these abundances are close to those inferred for the ambient cosmic radiation at 103 GeV, an energy where direct measurements are available. We interpret this as showing that the majority of the galactic sources are SNR, like Monogem, but of course with different ages and distances.
The search for confirmation
The identification of the peaks in figure 3 could be confirmed by searching for discontinuities in those entities that have given rise to estimates of the mean mass of the ambient cosmic radiation. However, such a search is bedevilled by two facts. First, it is in the nature of things that at any energy the mean mass of the single-source particles should be close to that of those injected for the ambient cosmic radiation. Second, the different analyses of the variety of EAS parameters used in deriving the mean masses give, notoriously, different results. Our conclusion, however, is that there is no evidence against our identifications.
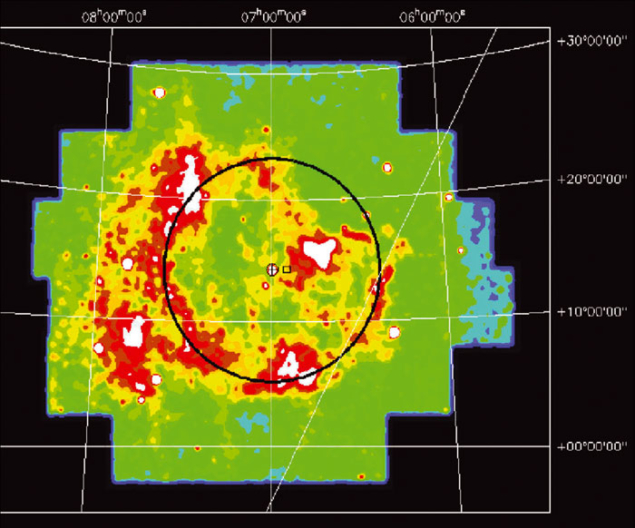
These differences provide a happy hunting ground for searches for changes with increasing energy in the nature of the interactions between cosmic rays and nuclei in the atmosphere. It must also be said, however, as we have pointed out, that recent results from CERN show no significant change in at least some of the interaction characteristics over the range 0.4–26 PeV. This is just where the cosmic-ray energy spectrum has its knee; LHC data on forward physics are eagerly awaited.