Six years after its construction began, the CNGS facility at CERN has sent its first batch of neutrinos 732 km to Gran Sasso in Italy in a highly successful commissioning run.
The CERN Neutrinos to Gran Sasso (CNGS) facility was built to create a neutrino beam to search for oscillations between muon-neutrinos and tau-neutrinos. An intense, almost 100% pure beam of muon-neutrinos is produced at CERN in the direction of the Gran Sasso National Laboratory (LNGS), almost 732 km away in Italy . There, the OPERA experiment is being constructed to find interactions of tau-neutrinos among those of other neutrinos.

The production of the CNGS beam of muon-neutrinos follows the “classic” scheme that was first used in the 1960s at Brookhaven and CERN, and has been refined ever since. An intense proton beam from CERN’s Super Proton Synchrotron (SPS) is sent to strike a target, in this case graphite. Protons that interact with nuclei in the target produce many particles, mostly unwanted, but including positively charged pions and kaons – mesons that decay naturally into pairs of muons and muon-neutrinos. Two magnetic lenses – the horn and the reflector – collect these mesons within a selected momentum range and focus them into a parallel beam towards LNGS. After a decay tube nearly 1 km long, all the hadrons – i.e. protons that have not interacted in the target, pions and kaons that have not yet decayed, and so on – are absorbed in a hadron stopper; only neutrinos and muons can traverse this solid block of graphite and iron. The muons, which are ultimately absorbed downstream in around 500 m of rock, are measured first in two detector stations. Only the neutrinos are left to travel onwards through the top layer of the Earth’s crust towards LNGS.
For the experimenters at LNGS, the beam’s key feature is the energy spectrum, as this determines the number of events that they can expect to measure. Two important energy-dependent ingredients have to be taken into account to maximize the number of tau-neutrino events that are anticipated: the probability for muon neutrino to tau-neutrino oscillation over the 732 km, and the probability for the tau-neutrino to leave a signal in a detector, i.e. the interaction cross-section for tau-neutrinos in matter, which is zero below a threshold of around 4 GeV. The product (convolution) of these two energy-dependent probabilities defines in effect an envelope in which the actual energy spectrum of the beam should fit. The graph below compares this convolution with the energy spectrum that was expected for the CNGS beam, as derived through Monte Carlo simulation, and shows how closely the match has been achieved. Note that the event rate at the OPERA detector at LNGS is very low. It will take many months of continuous CNGS running before the experiment can be expected to produce a neutrino energy spectrum like that in the graph below.
Six years in the making
CERN council approved the CNGS project in December 1999. Civil construction work began in September 2000 and was completed in June 2004. The underground work included the tunnel around 50–80 m below the surface for the 800 m proton beam line, as well as several caverns and access galleries. The facility uses protons from an extraction region at point 4 on the SPS, in common with the proton transfer line TI 8 for the Large Hadron Collider (LHC). A switch magnet at 100 m decides where the proton batches are sent: if the magnet is off, beam goes to the LHC, and if it is on, beam goes to the CNGS target. The beam line for CNGS then slopes down from the level of the SPS to a final slope of 5.6%, so that it points towards the LNGS.

While civil construction work continued, between July 2003 and April 2004 the beam dump (hadron stopper) and the 1 km decay tunnel were installed. Then in July 2004, with the construction work complete, an intense period of work began to install the electrical services, water-cooling and air-handling facilities. The overhead crane in the target chamber is an unusual feature that uses a rack-and-pinion system to cope with the slope of the tunnel. As well as being used in installation work, it will be needed for remote-handling in the harsh environment that is expected in the target chamber once the beam is operating at high intensity.
During the summer of 2005, installation of the services gradually gave way to the equipment installation in the proton beam tunnel as well as in the target chamber. By the end of November 2005, the proton beam was fully installed and the vacuum system closed, while work in the target chamber continued until spring 2006.
Testing, testing

During February to April of 2006, large parts of the CNGS facility closed as detailed tests of all components in the facility began. In particular, all of the 119 dipole and quadrupole magnets in the proton beam line were tested at nominal power and their polarities checked, and the water-cooling and ventilation systems were operated under nominal conditions. The control-system experts artificially introduced magnet faults in all of the elements to test in detail how the beam interlock system responded to such errors.
At the same time technicians performed exercises in which they completely changed the target and horn under realistic conditions, performing a large fraction of the work remotely, using the crane in the target chamber. The exercises allowed detailed log-sheets of every step to be established, recording the crane co-ordinates for the approach, picking-up, lifting, translating and depositing for every shielding block as well as for the target and horn systems.
Once the equipment experts had tested all of the CNGS components, it was time for the commissioning team to move to the CERN Control Centre (CCC). Using a wealth of computers and display screens, the team tested every aspect of the CNGS facility under the most realistic conditions – as if there was beam, but without beam. This was a stressful period for the controls group and colleagues in the SPS operations team who were writing software. However, they responded to the challenge and, as commissioning with beam later demonstrated, these dry runs meant that the systems were working, saving much valuable beam time.
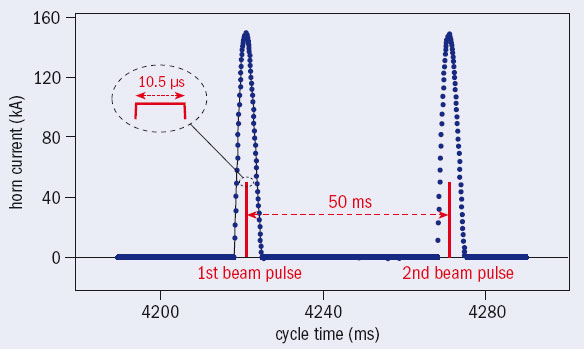
Large parts of the CNGS facility were closed on 19 May, in time for start-up with beam on 29 May. However, a last-minute schedule change, caused by a powering problem at the Proton Synchrotron, which feeds the SPS, implied that the first proton beam to CNGS could not be delivered until 10 July. This change of schedule allowed for another useful set of dry runs.
Beam commissioning begins
During the week of 10 July, the first of three CNGS beam commissioning weeks, the atmosphere in the SPS corner of the CCC was cheerful, but tension was nevertheless palpable. Initial tests of the extraction system with a CNGS-type beam had been done in autumn 2004, closely linked to the initial tests of the TI 8 beam. So it was no surprise to find that after only a few iterations, the kickers and septum magnets of the extraction channel from the SPS towards CNGS were well tuned, establishing a “golden trajectory”. On 11 July the first proton batch headed off to the CNGS target, and it was reassuring to see the proton beam well centred in all of the eight screens along the proton beam line.

The next step was to bring the beam position monitors (BPM) into operation. These important monitors were recuperated from the Large Electron–Positron collider, and equipped with sophisticated log-amp electronics, allowing them to measure the beam position rapidly and accurately. They revealed that the proton line was well tuned over its 800 m, with the maximum beam excursion far less than the permitted ±4 mm.
The CNGS commissioning also allows a valuable test for the Beam Interlock System that was developed for the LHC. The BPMs provide one of the crucial inputs to this system: any beam position that is more than 0.5 mm from the nominal trajectory creates an interlock to inhibit the next proton extraction and, in turn, provides an alarm to the SPS operations team. In addition, a series of beam loss monitors (BLMs) along the path of the protons measure tiny losses of protons, which would indicate that the beam is off course. Together, the BPMs and BLMs form a powerful means to protect the equipment in the CNGS proton beam line against damage from any losses larger than permitted by the very low thresholds in the system.
The beam size along the proton beam line was very close to the expectations from simulations. For a high-intensity beam – some 1013 protons for each extraction – the beam spot at the target was the expected 0.5 mm rms. The measured beam position stability is about 50 μm rms averaged over several days, and is much better than initially specified. Both the size and the stability of the beam are extremely important for protecting the target rods against rupture from the thermo-mechanical shock that is caused by the intense beam pulse: the beam size must not be too small (and hence concentrated) and the beam must hit the target close to the centre.

Much of the CNGS beam commissioning was done using a very low intensity proton beam – around a hundred times lower than the nominal value of 2.4 × 1013 protons for each extraction. This was necessary to protect the equipment from potential faults and other surprises. It was only during the last two days of commissioning that intensities reached the 1013 range. As a result of this economic use of the beam, less than 7 × 1015 protons were sent to CNGS during the entire commissioning phase, corresponding to about an hour of standard CNGS operation. In addition, while standard CNGS operation is foreseen with two 10.5 μs 400 GeV/c proton beam extractions for every SPS–CNGS magnet cycle, most of the commissioning work was done with one extraction only.
Lining up
The CNGS proton beam is directed at a graphite target. The target consist of 13 graphite rods 10 cm long and 9 cm apart; the first two rods are 5 mm in diameter, while the others rods are 4 mm in diameter. The rods need to be thin and interspaced with air to let high-energy pions and kaons that are produced at smaller angles fly out of the target without interacting again. This is important for the relatively high-energy neutrino beam at CNGS, as pions of higher energies decay in flight into neutrinos of higher energies. Beyond the target lie the magnetic focusing system comprising the horn and reflector. The two focusing systems are operated with a pulsed current of 150 kA for the horn and 180 kA for the reflector. Both horn and reflector are pulsed twice for each SPS cycle; the two pulses are separated by 50 ms, in-time with the two beam pulses.
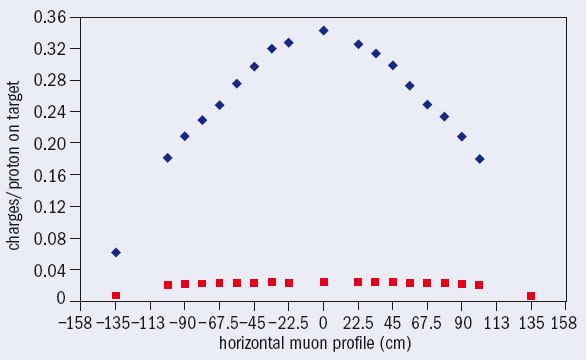
An important step during the beam commissioning was to cross-check the centring of the proton beam on the target. This is done by the Target Beam Instrumentation Downstream (TBID) monitor in which secondary electrons are produced by charged particles traversing a 145 mm diameter, 12 μm thick titanium sheet in a vacuum box. A beam scan across the target provides information on the maximum production of charged particles, in other words, on the best alignment of the proton beam with respect to the target.
The last check that can be made along the neutrino beam line is on the production of muons that are created in association with the muon-neutrinos in the decay of the pions and kaons produced in the target. Unlike the neutrinos, the muons are charged and can be rather easily detected, so during beam commissioning muon detector stations provided online feedback for the quality control of the neutrino beam. In CNGS these detectors must register up to 108muons for each cm2 in a very short pulse of 10.5 μs. This implies that the muons cannot be counted individually. So to monitor the muons CNGS uses nitrogen-filled, sealed ionization chambers. Such detectors have been used for many years, for example as BLMs around the SPS. CNGS users could take advantage of the most recent development of ionization chambers, which will be used as BLMs at the LHC. The first 76 of more than 3000 of these BLMs are now in use at CNGS. There are 37 fixed muon detectors in each of the two muon detector chambers. The monitors are arranged in a cross-shaped array to record permanently the horizontal and vertical profile. An identical motorized monitor is installed downstream of the fixed ones to allow cross-calibration of the fixed monitors and to probe the muon profile where there is no fixed monitor.
Muons passing through the monitors produce electron–ion pairs, which are collected on sets of electrodes that are 5 mm apart at 800 V. Each muon monitor has 64 electrodes over an active length of 345 mm. The signal recorded is the integral number of charges for each beam pulse. The CNGS beam commissioning team used the muon detector stations as an online feedback for the quality control of the neutrino beam. The measurement is in reasonably good agreement with the preliminary expectations based on the FLUKA simulation package.
• CERN funded the CNGS project with special contributions from Belgium , France (in kind, via LAL/IN2P3), Germany , Italy (INFN and Compania di San Paolo), Spain and Switzerland . The CNGS proton-beam magnets were built in Novosibirsk , within a collaboration agreement between the Budker Institute for Nuclear Physics, DESY and CERN. The CNGS facility has been constructed and the beam commissioned on schedule and within budget. We would like to thank the many colleagues involved in CNGS, who have worked hard to help make this project a success.