A recent workshop on the nucleon has pinpointed near to mid-term goals for QCD hadron structure physics. Nicola Bianchi and Rainer Jakob report.
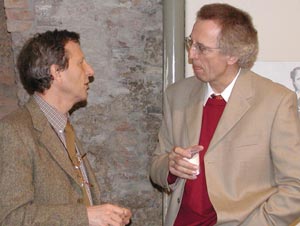
Exploration of the internal structure of the nucleon has reached a new stage. The international community of physicists studying hadron structure with electromagnetic probes has identified the main aims and goals for the near and mid-term future. This was the conclusion of the European Workshop on the QCD Structure of the Nucleon (QCD-N’02), which was held at the splendid Castello Estense in Ferrara, Italy, in April. Some 120 theorists and experimentalists reached a remarkable level of agreement on the hot new topics in the field, and on the avenues and strategies that need to be followed to unravel the inner structure of hadrons. This consensus has been translated into the “Declaration of Ferrara”, which has already been signed by many scientists interested in the future of hadronic physics.
Paola Ferretti Dalpiaz of Ferrara, Enzo De Sanctis of Frascati and Wolf-Dieter Nowak of DESY chaired the workshop, at which some 60 presentations focused on the issues of new distributions and fragmentation functions, generalized parton distributions and exclusive reactions, diffraction, nuclear effects and lattice quantum chromodynamics (QCD). A special session and a panel discussion, led by Peter Kroll of Wuppertal and Dirk Ryckbosch, spokesman of DESY’s HERMES experiment, was devoted to future facilities and measurements.
Hadronic origins
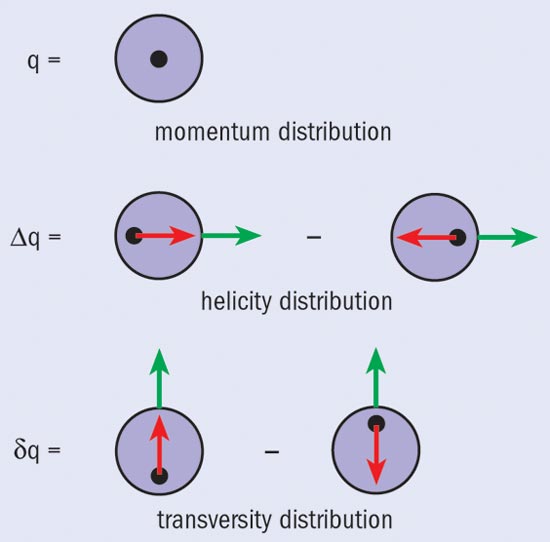
Several speakers emphasized the fact that the fundamental question of the origin of hadronic matter calls for a better understanding of the phenomenon of confinement in strong interactions. After all, only about 2% of the mass of the nucleon can be assigned to current quark masses, which are expected to be explained by the Higgs mechanism. The major part of the mass of hadrons is likely to originate from massless gluons – in other words from binding effects of strong interactions. The inner structure of nucleons, which make up most of the visible matter in the universe, as well as that of other hadrons, is still not understood from first principles in terms of quark and gluon degrees of freedom as described by the underlying quantum field theory, QCD.
The spin of the nucleon is a key issue in the investigation of its structure. It has been confirmed that the nucleon’s quark and antiquark constituents carry only 20-30% of its longitudinal spin. The rest is provided by the polarization of gluons and by the orbital angular momenta of quarks and gluons. First indications on the sign and size of the gluon polarization have been seen by the HERMES experiment, and precision measurements are on the way from COMPASS at CERN, and in the US from RHIC-spin at Brookhaven and E-161 at SLAC. However, another fundamental piece of the puzzle is still missing for the full picture – to complete our knowledge of nucleon spin we have to consider a situation where the nucleon spin is oriented perpendicularly to the direction of its motion. The associated distribution function is dubbed “transversity”, and has recently been the subject of major theoretical and experimental efforts. The difficulty with measuring transversity is related to its unusual spin property (it is a chirally odd function), which requires the occurrence of a second object with a similarly unusual spin property in an observable. It can be measured by looking at final-state hadrons in semi-inclusive experiments (where particles in the final state are studied), which involves additional fragmentation functions that help to describe certain spin-related aspects of hadronization. These new fragmentation functions are not only indispensable tools for the extraction of transversity, but are also of interest in themselves, since they bear witness to how confinement comes about. The use of the nuclear medium as a “fermiometer” to understand the scales and the dynamics of the hadronization processes of quarks was pointed out at the workshop. Information from the clean process of lepton-nucleus scattering is also needed for a better understanding of the hadron yield and spectra produced in heavy-ion reactions.
The complexity of the task of mapping transversity requires the interplay of different and highly complementary measurements. A strong combined effort in this direction was presented as a major part of the programme of HERMES, COMPASS, RHIC-spin and BELLE at Japan’s KEK laboratory.
Transverse localization
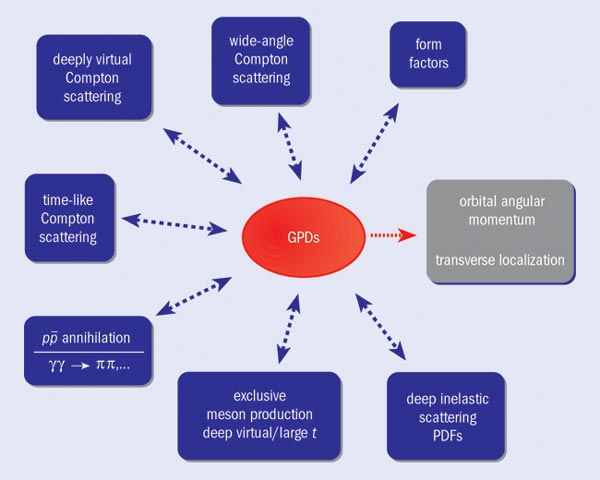
Physicists have realized in the last few years that there is more to learn about nucleon structure from exclusive reactions. The advent of new fundamental and conceptual ideas, the so-called generalized parton distributions (GPDs), has triggered enormous theoretical and experimental activity. GPDs provide a unified description of exclusive (where all produced particles are studied in conjunction with the incident particle) and inclusive (averaged over all final states) hard reactions. Moreover, the formalism of GPDs has a sound basis in QCD and relies on formal factorization theorems.
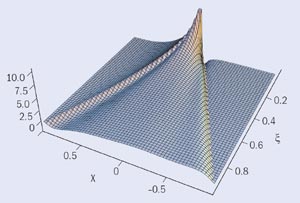
Discussion on GPDs was initiated by the exciting possibility of accessing the orbital angular momentum contribution of the nucleon’s spin, which is so far completely unknown. A qualitative new feature of GPDs is that they allow insight into the transverse structure of the nucleon. Inclusive deep inelastic scattering processes probe the ordinary momentum distribution of the nucleon. Exclusive reactions, on the other hand, allow the distribution to be probed as a function of the distance of a quark or gluon from the centre of mass of the nucleon. In other words, this effectively enables nucleon tomography, since by combining information from different measurements the nucleon can be scanned in transverse slices.
Encouraging first results along these lines from the H1, ZEUS and HERMES experiments at DESY, and from CLAS at Jefferson Lab in the US were presented at the workshop. These are in general agreement with more recent GPD expectations, and so have demonstrated the feasibility of proposed future measurements. Moreover, the emerging interest to link the classical diffractive description of exclusive processes at high energy with the GPD description of exclusive processes will require precise measurements over a broad kinematic range.
Moments of the transversity distribution and of the generalized parton distributions are among the quantities amenable to lattice gauge calculations. For example, results on the lowest moment of transversity, the so-called tensor charge, were reported. The strongly QCD-inspired instanton model calculations also provide verifiable predictions. These are very promising ways to link phenomenological observations to first principle theoretical considerations.
The Declaration of Ferrara
After presentations of current exploratory studies and discussions of new ideas, workshop participants concluded that future dedicated facilities are needed. This led to the view of a large community of European hadron structure researchers being expressed in the Declaration of Ferrara.
It has become clear that in-depth studies of the energy, momentum and spin-dependence of exclusive cross-sections cannot be performed with the current generation of accelerators and spectrometers, but require substantial advances in experimental facilities and techniques. This is mostly due to luminosity, duty-cycle and kinematic resolution limits, since the most interesting reactions are rare and have large backgrounds. High experimental precision can only be accomplished with luminosities of at least 1035 cm-2s-1, requiring an accelerator with a duty cycle of 10% or more. Beam energies of 25-50 GeV are needed to cover a kinematic range suitable for extracting cross-sections and their scale-dependence in exclusive measurements. For non-exclusive studies of hadron structure, the optimal beam-energy range is 50-100 GeV. The highest possible polarization of beam and targets is required in both cases. Large-acceptance detector systems with high-rate capabilities and with a mass resolution of the order of a third of the pion mass, essential for the measurement of exclusive channels, will also lead to a new quality of meson and baryon spectroscopy in lepton-nucleon scattering.
Several scenarios for a new generation of precision experiments to study QCD with electromagnetic probes are being studied in Europe and the US. Collider and fixed-target options are fully complementary, since the former cover a wider kinematic range and the latter provide considerably higher luminosities. The fixed-target option, favoured by European scientists, can be technically realized in a cost-effective way based upon the technology being developed for future linear colliders, especially if existing or projected infrastructure can be used.
The conclusion of the workshop was that in order to keep Europe’s leading role in studying hadron structure and QCD at all scales with energetic electromagnetic probes, a new fixed-target facility with a high duty cycle providing polarized beams in the energy range 25-100 GeV is needed.