Workshops on next-generation neutrino detectors and devices to detect possible nucleon decay have been held since 1999. Jacques Bouchez of the French atomic energy commission (CEA) reports on the most recent one, which was hosted by CERN in January.

Great achievements have been made in recent years using the very large underground detectors initially designed to observe possible proton decays. The pioneers in this field were KOLAR in India, IMB in the US, Kamiokande in Japan, and NUSEX and Fréjus in Europe, the largest of which used an instrumented mass of the order of 1 kiloton. Through the non-observance of proton decays it was possible to reject the simpler versions of grand unification theories (which unify the weak, strong and electromagnetic interactions under the same theory). However, the actual results harvested were far more plentiful – a detailed study of atmospheric neutrinos (produced by cosmic rays in the Earth’s atmosphere), which constituted the main background of the sought-after decays, provided hints of an anomaly in the flavour composition of these neutrinos. These measurements have since been refined by the 50 kiloton Superkamiokande detector, which has unambiguously established the existence of flavour oscillations, where muon neutrinos transform into tau neutrinos.
In 1987 the Kamiokande and IMB detectors, both water Cerenkov counters with an energy threshold of only a few MeV (made possible by the high level to which the water was purified), observed a small burst of neutrinos coming from supernova 1987A. This observation opened the way for neutrinos to be used as the messengers of the universe, an avenue since pursued by experiments such as AMANDA at the South Pole and ANTARES, which is currently being deployed in the Mediterranean Sea. Thanks to their very low energy thresholds, Kamiokande and Superkamiokande have also been able to measure the neutrino flux from the Sun and confirm that a large deficit exists, which also hints at neutrino oscillation. This interpretation was confirmed in spectacular fashion in June 2001 and reinforced this April by results from the Sudbury Neutrino Observatory’s unique 1 kiloton heavy water Cerenkov detector in northern Ontario, Canada (Direct evidence seen for oscillations), bringing positive evidence that neutrinos have mass.
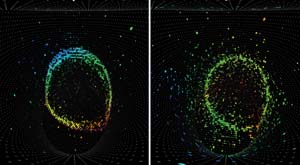
These advances have led physicists to consider the possibilities offered by detectors not of 1 or 50 kilotons but of 1 megaton. Such devices would be capable of detecting proton decays at the very weak rates predicted by the latest supersymmetric grand unification theories. They would allow the tens of thousands of neutrino interactions produced by a supernova in our galaxy to be observed in a matter of seconds, thus supplying a wealth of information on explosion mechanisms. Such detectors could also act as targets for the neutrino superbeams currently being studied in Japan, the US and Europe.
A workshop on megaton detectors was held at CERN in January to take stock of developments in this field. It brought together 65 participants from Europe, 25 from America and 10 from Asia. The speakers addressed three main themes in detail – proton decay, supernovae and superbeam-assisted neutrino oscillations – from both theoretical and experimental points of view. They described very large Cerenkov detector projects and the underground sites that would potentially house them. Japanese, American and French engineers discussed the problems associated with digging deep underground cavities with a volume of 1 million m3. Suggestions were also made for alternatives to the Cerenkov technique. These included a liquid-argon detector along the lines of the ICARUS detector being prepared for Italy’s Gran Sasso underground laboratory, but with a mass of up to 100 kilotons, and the specialized OMNIS detector, for which a UK-US collaboration proposes a lead target for supernova neutrinos. Fine-grained calorimetric detectors that may be envisaged for high-energy neutrino superbeams were also discussed.
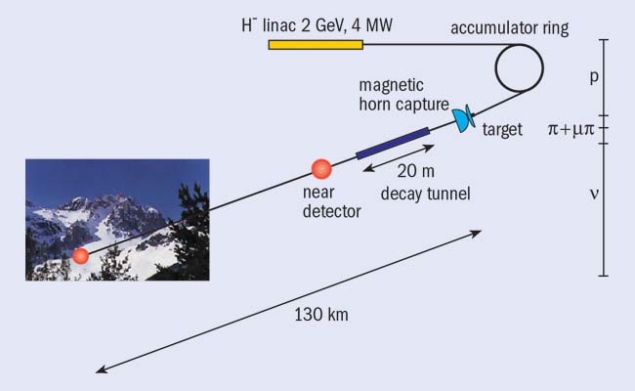
On the experimental side, the workshop brought together two communities of physicists – those interested in neutrinos and those interested in non-accelerator physics – which now require similar types of detector. The convergence of several themes around a single detector capable of addressing them all can only strengthen these ambitious projects, which require worldwide collaborations. The theoretical implications of both proton decay research and a fine study of neutrino mixing are of fundamental importance as they open up windows beyond the Standard Model. These subjects can tell us about the structure of grand unification theories and baryogenesis, topics that must be understood to explain the domination of matter over antimatter in our universe.
As far as proton decay is concerned, in the e+p0 decay channel a 500 kiloton water Cerenkov detector can provide a sensitivity of 1035 years for the lifetime of the proton, compared with the current limit of the order of 5 ¥ 1033 years. The K+n decay channel, favoured in certain scenarios, is a more difficult task for water Cerenkov detectors, and for this decay a more ambitious 100 kiloton liquid-argon detector could achieve a similar sensitivity.
With megaton detectors, the search for supernovae can be spread to neighbouring galaxies (we would observe some 20 neutrinos for an explosion in the Andromeda galaxy), and this has the virtue of increasing the rate of visible explosions to one every 10 years.
Neutrino superbeams
The most recent subject of study concerning the potential of megaton detectors relates to neutrino superbeams. These are similar to current neutrino beams in their method of production, but will use much more intense primary proton beams. Such proton supermachines are currently being considered for a range of applications including hybrid nuclear reactors and waste reprocessing plants, spallation neutron sources, intense sources of radioactive nuclei, and as the first component of a neutrino factory or a muon collider. These are low-energy machines (at the GeV scale) with about 1 MW of power (compared with several hundred kilowatts at machines such as CERN’s SPS). These machines could supply superbeams of unrivalled intensity for neutrino research.
Today we know that neutrinos have a mass and that they mix, giving rise to the oscillation phenomenon. But there is one oscillation we have yet to observe – that which links muon and electron neutrinos with the frequency observed for atmospheric neutrinos. This oscillation is weak since it is governed by a mixing angle (q13) that, thanks to the French CHOOZ reactor-based experiment, we know to be lower than 10°. The entire neutrino factory programme hinges on the existence of this tiny oscillation. Neutrino superbeams will therefore be used to demonstrate its existence for q13 values down to 1°. Such an observation would be reassuring before the construction of a neutrino factory can be launched.
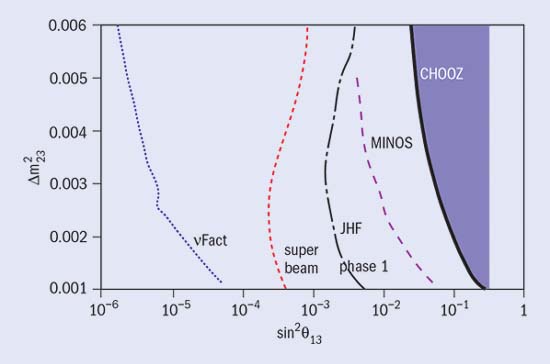
To this end, the Japanese will soon have a 0.8 MW proton accelerator at the Japan Atomic Energy Research Institute, and in 2007 plan to send a neutrino superbeam to the Superkamiokande detector 300 km away. A sensitivity of 2.4° for q13 is expected. To get the sensitivity down to 1°, Japanese physicists envisage increasing the proton power to 4 MW after 2012 and building a 1 megaton detector, Hyperkamiokande, on the Kamioka site.
In Europe, CERN’s superconducting proton linac (SPL) project aims to supply 2.2 GeV protons at 4 MW by 2012. The optimal baseline for studying the oscillation in question corresponds to the distance between CERN and the Fréjus laboratory. The forthcoming construction of a gallery parallel to the existing road tunnel at Fréjus provides the opportunity to dig a cavern capable of housing a megaton detector comparable to the UNO underground nucleon decay and neutrino observatory being designed in the US. Such a project would provide a sensitivity of 1° on q13. The SPL would also allow long-lived radioactive nuclei to be produced and stored in storage rings whose straight sections, aimed at the Fréjus underground site, would supply electron-neutrino beams that, in conjunction with the muon-neutrino superbeam, would open up the possibility of studying time-reversal symmetry-breaking in the neutrino sector.
US scientists are looking into using the beam that will be sent from Fermilab to the MINOS detector in 2005 and increasing the proton power from 0.4 to 1.6 MW. A superbeam is also being considered at Brookhaven. It will still be necessary to dig a cavity to house a very large detector, and this could be done at the Homestake mine (which has recently been transferred to the Department of Energy) or elsewhere. The current Fermilab beam will provide a sensitivity of 4° on q13 using a 5-20 kiloton detector. Future projects could lower this limit to around 1.5° by using either UNO or a 70 kiloton liquid-argon detector.
Looking to the future

Although none of these plans has yet been approved, it seems likely that such a project will be realized. It will form a key component of the global fundamental physics infrastructure of the future, and its location remains to be decided. Europe has a strong track record in the field, but the Japanese project, in its first phase using the Superkamiokande detector, is currently the most advanced. The workshop held at CERN provided the opportunity for a first round of discussions between Europeans, Americans and Japanese with a view to worldwide collaboration around this Japanese first phase. If no oscillation is discovered it will then be necessary to move into the megaton phase, and the siting of such a detector is, for the moment, an entirely open question. Multipurpose megaton detectors will address many open physics issues, and promise, in parallel to CERN’s Large Hadron Collider and the electron-positron linear colliders, to provide exciting results in the decades to come.