In August nearly 700 scientists and engineers from North America, Asia and Europe got together at Snowmass in the US to advance the design of the International Linear Collider and its detectors, and to refine the physics case for this next-generation machine.
In August 2004 the Executive Committee of the American Linear Collider Physics Group (ALCPG), galvanized by the technology choice for the future International Linear Collider (ILC), decided to host an extended international summer workshop to further the detector designs and advance the physics arguments. Subsequently, the International Linear Collider Steering Committee (ILCSC) elected to hold their Second ILC Accelerator Workshop in conjunction with the Physics and Detector Workshop. Ed Berger of Argonne and Uriel Nauenberg of Colorado were selected to co-chair the organizing committee for this joint workshop, which was held at Snowmass, Colorado, US, for two weeks in August. ALCPG co-chairs Jim Brau of Oregon and Mark Oreglia of Chicago, along with accelerator community representatives Shekhar Mishra from Fermilab and Nan Phinney from SLAC, rounded out the committee. While hosted by the North American community, the workshops were planned with worldwide participation in all the advisory committees and in the scientific programme committees for the accelerator, detector, physics and outreach activities.
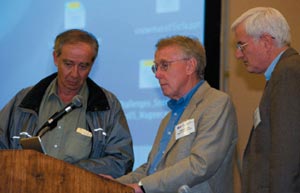
As Berger described in the opening address, the primary accelerator goals at Snowmass were to define an ILC Baseline Configuration Document – to be completed by the end of 2005 – and to identify critical R&D topics and timelines. On the detector front, the goal was to develop detector design studies with a firm understanding of the technical details and physics performance of the three major detector concepts, the required future R&D, test-beam plans, machine-detector interface issues, beamline instrumentation and cost estimates. The physics goals were to advance and sharpen ILC physics studies, including precise higher-order calculations, synergy with the physics programme of CERN’s Large Hadron Collider (LHC), connections to cosmology, and, very importantly, relationships to the detector designs. A crucial fourth goal was to facilitate and strengthen the broad participation of the scientific and engineering communities in ILC physics, detectors and accelerators, and to engage the greater public in this exciting work.
A rich new world
Over the past few years, prestigious panels in Europe (the European Committee for Future Accelerators – ECFA), Asia (the Asian Committee for Future Accelerators – ACFA) and the US (the High Energy Physics Advisory Panel – HEPAP) have reached an unprecedented consensus that the next major accelerator for world particle physics should be a 500 GeV electron-positron linear collider with the capability of extension to higher energies. This machine would be ideal for exploiting the anticipated discoveries at the LHC and would also have its own unique discovery capabilities. The ability to control the collision energy, polarize one or both beams, and measure cleanly the particles produced will allow the linear collider to zero in on the crucial features of a rich new world that Peter Zerwas of DESY described on the first day of the workshop, which might include Higgs bosons, supersymmetric particles and evidence of extra spatial dimensions.
This physics programme dictates specific requirements for the detectors and for the accelerator design. As the ILC community turns increasingly to design and engineering, there was considerable activity in the physics groups to formulate these requirements concretely. Early in the workshop, an international panel set up this spring presented a proposed list of benchmark processes to be used in optimizing the ILC detector designs. This brought a new flavour to the physics discussions – one that will continue in future work on physics at the ILC.

This influence was felt most strongly in the working groups on Higgs physics and supersymmetry. Precision electroweak data predict that the neutral Higgs boson will be observed within the initial energy reach of the ILC, which will provide a microscope to study the whole range of possible Higgs boson decays and measure coupling strengths to the percent level. To accomplish this goal, the ILC detectors must have significantly better performance in several respects than those at CERN’s Large Electron-Position collider (LEP). In contrast with the quite specific implications of Higgs boson physics, the idea of supersymmetry encompasses various models with diverse implications. Some of the signatures of supersymmetry will be studied at the LHC, but the problem of understanding the exact nature of any new physics will be a difficult one. Through the study of a diverse set of specific parameter sets for supersymmetry, work done at Snowmass showed that the ILC experiments could address this problem robustly, and the necessary detector performances were specified.
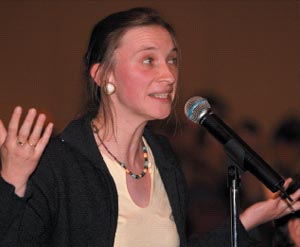
Precision is crucial
The precision of the ILC experiments should be supported by equally precise theoretical calculations. Among those discussed at the workshop were Standard Model analyses, including higher-order contributions in quantum chromodynamics, calculations of radiative corrections to the key Higgs boson production processes, and precision calculations within models of new physics. The Supersymmetry Parameter Analysis project, presented at Snowmass, proposes a convention for the parameters for supersymmetry models from which observables can be computed to the part-per-mille level for unambiguous comparison of theory and experiment. The fourth in the series of LoopFest conferences on higher-order calculations took place during the Snowmass workshop, the highlight this year being a presentation of new twistor space methods for computing amplitudes for emission of very large numbers of gluons and other massless particles. New calculations of the process e+e− → tbar th showed that higher-order corrections enhance this process by a factor of two near threshold, making it possible for the 500 GeV ILC to obtain a precise measurement of the top quark Yukawa coupling.

The capabilities of the ILC will make it possible to explore new models, which include Higgs sectors with CP violation (for which the ILC offers specific probes of quantum numbers), and models with a “warped” extra dimension, which predict anomalies in the top quark couplings that can be seen in tbar t production just above threshold.
Many of the discussions of new physics highlighted the connections to current problems of cosmology. Supersymmetry and many other models of new physics contain particles that could make up (at least part of) the cosmic dark matter. If these models are correct, dark-matter candidates will be produced in the laboratory at the LHC. Studies at Snowmass showed how precise measurements at the ILC could be used to verify whether these particles have the properties required to account for the densities and cross-sections of astrophysical dark matter. Here all the strands of ILC physics – exotic models, precision calculations and incisive experimental capabilities – could combine to provide physical insight that can be obtained in no other way.
The accelerator design effort
In August 2004 the International Technology Recommendation Panel concluded that the ILC should be based on superconducting radio-frequency accelerating structures. This recommendation has been universally adopted as the basis for the ILC project, now being coordinated via the Global Design Effort (GDE), led by Barry Barish from Caltech. At Snowmass, the accelerator experts carried the baton from the successful launch of the ILC design effort at the first ILC workshop at KEK in Japan in November 2004. Snowmass also provided the forum for the first official meeting of the GDE. The working groups established for the first ILC workshop at KEK formed the basis of the organizing units through Snowmass. In addition, six global groups were formed to work towards a realistic reference design: Parameters, Controls & Instrumentation, Operations & Availability, Civil & Siting, Cost & Engineering, and Options.
Sources of electrons and positrons are the starting points of the accelerator chain. The successful production of intense beams of polarized electrons at the SLAC Linear Collider (SLC) between 1992 and 1998 demonstrated the best mechanism for producing electrons. When polarized laser light is fired at special cathode materials, electrons are produced with their spin vectors aligned, with polarization of up to 90% achieved in the laboratory. The ability to select the “handedness” of the beam is an incisive capability that will allow probes of the left- or right-handed nature of the couplings of new particles, such as those in supersymmetric models.
As well as the positron production systems used previously, other approaches are being studied to achieve polarized beams. One involves passing the high-energy electron beam through the periodic magnetic field provided by an “undulator”, similar to those used at synchrotron light sources. The intense photon beams radiated by the undulating electrons can be converted in a thin target into electron-positron pairs. A second method involves boosting the energies of photons produced in laser beams by Compton back-scattering them from electrons, and then similarly converting the boosted photons to yield positrons. If the intermediate photons are polarized, both of these methods allow polarized positron production.
The electron and positron beams produced must be “cooled” in so-called damping rings, in which their transverse size is reduced via synchrotron radiation during several hundred circuits. A few different designs are being studied for these rings. Challenges include precise component alignment and the high degree of stability required for low emittance, while minimizing collective effects that can blow up the beams.
Most of the length of the linear collider, some 20 km or so, will be devoted to accelerating the electron and positron beams in two opposing linacs. The debate at Snowmass centred on critical issues, such as the operating choice for the accelerating voltage gradient in the superconducting niobium cavities and the choice of advanced technologies that must be used to power the cavities. The details of the shape and surface preparation of the cavities are among the issues that affect the gradient that can be supported. Larger radii of curvature of the cavity lobes are desirable to reduce peak surface electric fields that can induce breakdown. Also, advanced surface preparation techniques such as electropolishing are being refined, and cavities are being produced and tested by strong international teams at regional test facilities. Based on experience to date, a draft recommendation was reached for a mean initial operating gradient of around 31 MV per metre. Each linac would then need to be just over 10 km long to reach the initial target centre-of-mass energy of 500 GeV.
Similar expert attention was devoted to the modulators, klystrons and distribution systems that convert “wall-plug” power into the high-power (10 MW) millisecond-long pulses applied to the cavities. Industrial companies in Europe, Asia and the US have developed prototype klystrons for this purpose. These are in use at the TESLA Test Facility at DESY, which provides a working prototype linac system. Several innovative ideas for solid-state modulators or more compact klystrons are also being explored with industry.
Once at their final energy, the beams must be carefully focused and steered into collision. The collision point lies at the ends of the two linacs and encompasses the interaction region, including the detector(s). The working recommendation, defined at the workshop at KEK, is to consider two interaction regions, each with one detector. Many important ramifications were discussed at Snowmass. For example, the current plan calls for the beams to be brought into the interaction region with a small horizontal crossing angle of either 2 or 20 mrad. In either case the final-focus magnets must be carefully designed to be compact and stable with respect to vibrations that could be transferred to beam motion. A detailed engineering design is being prepared, which will also include beam-steering feedback systems to maintain the beams in collision and optimize the luminosity. Intermediate values for the crossing angle, such as 14 mrad, are also under study.
Of no less importance is the need to remove the spent beams safely from the interaction region and transport them to the beam dumps. As each beam carries an equivalent of several megawatts of power, the design must allow the necessary clearances, and be capable of being aborted safely in the event of equipment failure. The “machine protection” system and beam dumps remain subjects for active R&D. Many crucial diagnostic systems for measuring the beam energy, polarization and luminosity will be based in the extraction lines, and excellent progress was made in defining the locations and configurations of the necessary instrumentation.
The GDE will build on the consensus reached at Snowmass and produce an accelerator Baseline Configuration Document (BCD) by the end of 2005. As Nick Walker from DESY summarized at the end of the workshop, the BCD will define the most important layout and technology choices for the accelerator. For each subsystem a baseline technology will be specified, along with possible alternatives which, with further R&D, will offer the promise to reduce the cost, minimize the risk or further optimize the performance of the ILC. The engineering details of the baseline design will then be refined and costed. A Reference Design Report will follow at the end of 2006. This will represent a first “blueprint” for the ILC, paving the way for a subsequent effort to achieve a fully engineered technical design.
Detector concepts
The Snowmass workshop was an important opportunity for proponents of the three major detector-concept studies to work together on their detector designs. They are planning to draft detector outline documents before the next Linear Collider Workshop (LCWS06) in Bangalore in March 2006. Detector capabilities are challenged by the precision physics planned at the ILC. The environment is relatively clean, but the detector performance must be two to ten times better than at LEP and the SLAC Linear Collider. Details of tracking, vertexing, calorimetry, software algorithms and other aspects of the detectors were discussed vigorously.
The three major international detector concepts rely on a “particle flow” approach in which the energy of jets is measured by reconstructing individual particles. This technique can be much more precise than the purely calorimetric approach employed at hadron colliders like the LHC. In a typical jet, 70% of the energy consists of hadrons, which are measured with only moderate resolution in the hadron calorimeter, while 30% consists of photons, which are measured with much better precision in the electromagnetic calorimeter. Of the hadronic energy typically 60% is carried by charged particles, which can be measured precisely with the tracking system. The hadron calorimeter is thus relied on only for the 10% carried by neutral hadrons. For the particle-flow approach, it is necessary to separate the charged and neutral particles in the calorimeters, where the showers overlap or are often very close to each other. Separation of the showers is accomplished differently in each of the detector concepts, trading off detector radius, magnetic-field strength and granularity of the calorimeter.
Physical processes to be studied at the ILC require tagging of bottom and charm quarks with unprecedented efficiency and purity, as well as of tau leptons.
A specialized group worked on the development of the particle-flow algorithms. A conventional shower-reconstruction algorithm tends to combine the showers of different hadrons, but more sophisticated software should be able to separate them based on the substructure of the showers. At present the energy resolution of jets is still limited by confusion in the reconstruction, but significant progress was achieved at Snowmass, with optimism that a resolution of 30%⁄√E can be reached.
In the Silicon Detector Concept (SiD) the goal is a calorimeter with the best possible granularity, consisting of a tungsten absorber and silicon detectors. To make this detector affordable, a relatively small inner calorimeter radius of 1.3 m is chosen. Shower separation and good momentum resolution are achieved with a 5 T magnetic field and very precise silicon detectors for charged particle tracking. The fast timing of the silicon tracker makes SiD a robust detector with respect to backgrounds.
The Large Detector Concept (LDC), derived from the detector described in the technical design report for TESLA, uses a somewhat larger radius of 1.7 m. It also plans a silicon-tungsten calorimeter, possibly with a somewhat coarser granularity. For charged particle tracking, a large time-projection chamber (TPC) is planned to allow efficient and redundant particle reconstruction. The larger radius is needed to achieve the required momentum resolution.
The GLD concept chooses a larger radius of 2.1 m to take advantage of a separation of showers just by distance. It uses a calorimeter with even coarser segmentation and gaseous tracking similar to the LDC. Progress at Snowmass on the GLD, LDC and SiD concepts was summarized at the end of the workshop by Yasuhiro Sugimoto of KEK, Henri Videau of Ecole Polytechnique and Harry Weerts of Argonne, respectively. A fourth concept was introduced at Snowmass, one not relying on the particle-flow approach.
A common challenge for all detector concepts is the microvertex detector. Physical processes to be studied at the ILC require tagging of bottom and charm quarks with unprecedented efficiency and purity, as well as of tau leptons. This task is complicated by backgrounds from the interacting beams and the long bunch trains during which readout is difficult. The detectors must be extremely precise, and also extremely thin, to avoid deflection of low-momentum particles and deterioration of interesting information. Several technologies are under discussion, all employing a “pixel” structure based on the excellent experience of the SLD vertex detector at SLAC, ranging from charge-coupled devices (CCDs) and complementary metal-oxide semiconductor (CMOS) sensors used in digital cameras, to technologies that improve on the ones already used for the LHC.
In the gaseous tracking groups much discussion centred on methods to increase the number of points in the TPC, compared with LEP experiments. A possibility is to use gas electron-multiplier foils, a technology that was developed at CERN for the LHC detectors. Micromesh gaseous structure detectors, or “micromegas”, are another option, pursued mainly in France. The availability of test beams is crucial for advancing detector designs. TPC tests have been performed at KEK, and a prototype of the electromagnetic calorimeter has been tested at DESY. Further tests are also planned at the test-beam facilities at CERN and Fermilab.
As befitting a workshop with both detector and accelerator experts present in force, discussion of the machine-detector interface issues played a big role. The layout of the accelerator influences detectors in many ways – for example, beam parameters determine the backgrounds, the possible crossing angle of the beams affects the layout of the forward detectors, and the position of the final focus magnets dictates the position of important detector elements. All of these parameters have to be optimized by accelerator and detector experts working in concert. At a well attended plenary “town meeting” one afternoon, several speakers debated “The Case for Two Detectors”. Issues included complementary physics capabilities, cross-checking of results, total project cost and two interaction regions versus one.
Outreach, communication and education
A special evening forum on 23 August addressed “Challenges for Realizing the ILC: Funding, Regionalism and International Collaboration”. Eight distinguished speakers, representing committees and funding agencies with direct responsibility for the ILC, shared their wisdom and perspectives: Jonathan Dorfan (chairman of the International Committee for Future Accelerators, ICFA), Fred Gilman (HEPAP), Pat Looney (formerly of the US Office of Science and Technology Policy), Robin Staffin (US Department of Energy), Michael Turner (US National Science Foundation), Shin-ichi Kurokawa (ACFA chair and incoming ILCSC chair), Roberto Petronzio (Funding Agencies for the Linear Collider) and Albrecht Wagner (incoming ICFA chair). The brief presentations were followed by animated questions and comments from many in the audience.
Educational activities played a prominent role in the Snowmass workshop. Reaching out to particle experimenters and theorists, the accelerator community ran a series of eight lunchtime accelerator tutorials. Of broader interest to the general public were a dark-matter café and quantum-universe exhibit in the Snowmass Mall, a Workshop on Dark Matter and Cosmic-Ray Showers for high-school teachers, and a cosmic-ray-shower study in the Aspen Mall. Two evening public lectures attracted many residents and tourists, with Young-Kee Kim talking on “E = mc2“, and Hitoshi Murayama on “Seeing the Invisibles”. A physics fiesta took place one Sunday in a secondary school in Carbondale, where physicists and teachers from the workshop engaged children in hands-on activities.
Communication was also on the Snowmass agenda, and the communications working group defined a strategic communication plan. During the workshop a new website, www.linearcollider.org , was launched together with ILC NewsLine, a new weekly online newsletter open to all subscribers.
• Proceedings of the Snowmass Workshop will appear on the SLAC Electronic Conference Proceedings Archive, eConf.