Energy loss through synchrotron radiation is the bane of circular high-energy electron accelerators, which is one reason why CERN’s next accelerator, the Large Hadron Collider will be accelerating protons. Nevertheless, synchrotron radiation at the Large Hadron Collider still has to be considered carefully.
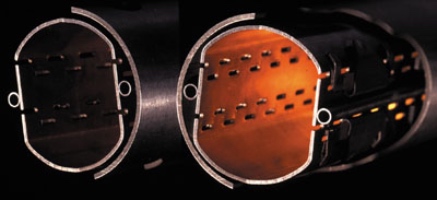
As a beam of charged particles is bent in a magnetic field, the particles lose energy by synchrotron radiation. The amount of energy lost is far greater for light particles than for heavy ones. Protons, the particles to be accelerated in CERN’s forthcoming Large Hadron Collider (LHC), are some 2000 times as heavy as the electrons and positrons of the laboratory’s current LEP accelerator, thus they lose far less of their energy to synchrotron radiation.
However, although the proportion of energy lost at the LHC will be much less than at LEP, the number of photons emitted by beam particles will, surprisingly, be much greater. Moreover, these photons will give rise to a number of undesirable effects that must be carefully controlled in a cryogenic vacuum environment. They will stimulate the release of gas molecules trapped in the walls of the LHC’s vacuum chamber, they will cause the emission of electrons (photo-emission), that in turn may liberate secondary electrons, and these will cause heating.
Beam screen solution
A partial solution will come in the form of beam screens that are designed to prevent the LHC’s bending magnets from overheating and losing their superconductivity. These metallic tubes lining the LHC vacuum chamber will be held in the 5-20 K range, whereas the magnets will operate at just 1.9 K. The beam screens will carry away heat generated by so-called image currents, induced by the charge of the beams, as well as by the synchrotron radiation.
However, the story doesn’t end there. Within the LHC’s bending magnets, synchrotron radiation will tend to strike the outside wall of the beam screen along a very tight band. Electrons liberated by this radiation will immediately be turned back by the magnetic field and reabsorbed. The only problem this will cause is heating of the beam screen, which is easily dealt with.
However, if a photon is reflected and strikes the roof or floor of the beam screen, liberating electrons there, these electrons will be accelerated in a spiralling path by the positive charge of the passing proton bunch towards the opposite wall (bottom or top) of the beam screen, where they may release more electrons. These in turn will be attracted by the following proton bunch and may be accelerated back to the top or bottom, releasing more electrons in a potential runaway situation. This is called multipacting, a phenomenon that is familiar to designers of accelerating cavities.
With the LHC, however, multipacting will not be quite the problem it may at first seem. The process is self-damping to a certain extent because, when an electron cloud has formed close to the walls of the beam screen, it inhibits further electron emission. Keeping this cloud manageable, however, is a fine art. Calculations suggest that, as long as the maximum number of secondary electrons produced per primary electron is less than 1.3, then runaway will not occur.

Unfortunately, the current beam screens give a coefficient of around 2.4, so work is under way to finetune the design. Some improvement will occur naturally as the beam screens become conditioned by exposure to synchrotron radiation. But to reach the levels required at the LHC demands an in-depth understanding of factors such as the reflectivity of the emitted photons, the primary and secondary electron yields and the amount of gas release stimulated by electrons and photons.
In 1996 a group of researchers in CERN’s LHC division began a study of these quantities using both synchrotron radiation from the Electron Positron Accumulator (normally used to collect particles for LEP) and a laboratory electron source. The group’s goal is to optimize the design of the beam screens by seeing how different manufacturing techniques influence their behaviour on exposure to synchrotron radiation. The current design is a 50 µm layer of copper, chosen for its low electrical resistivity, covering a rigid structure of high manganese steel, chosen for its low magnetic susceptibility. Low resistivity is important in allowing the image currents induced by the LHC’s protons to move as freely as possible to keep resistive heating to a minimum.

Three beam-screen models have been tested at room temperature: a mirror-like copper laminate, electroplated copper and a sawtooth form. The results from the tests suggest that there may be some advantages with the sawtooth design, but further investigations are necessary to be sure that there are no unforeseen drawbacks with it.
The next step is to study beam screen behaviour at cryogenic temperatures. Last year the group began COLDEX (cold experiment). This was designed to measure synchrotron radiation-induced gas release, but it is ideal for all the other measurements as well. When LEP shuts down in November, the COLDEX apparatus will be inserted into the EPA ring to investigate how LHC beam screens operate with positively charged beams passing through them. The results should help to ensure that the LHC’s electron clouds have, if not a silver lining, at least a copper one.