First results from a novel experiment at CERN.
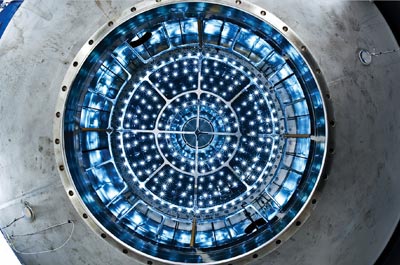
Studies of the effects on clouds of atmospheric ions from galactic cosmic rays extend as far back as C T R Wilson at the beginning of the 20th century, whose work on simulating ion-droplet processes led to a Nobel Prize in Physics for his development of the cloud chamber. Some 50 years later, laboratory studies beginning in the 1960s established ion-enhancement of aerosol nucleation at ion-production rates that are characteristic of the lower atmosphere (e.g. Vohra et al. 1984). Aerosols are tiny liquid or solid particles suspended in the atmosphere and – above a size of around 100 nm – they provide the seed particles for all cloud droplets; “nucleation” indicates that they are produced by the clustering (condensation) of trace atmospheric molecules rather than by direct emission into the atmosphere, such as sea-spray particles.
Robert Dickinson of the National Center for Atmospheric Research, Boulder, Colorado, was the first to postulate in detail a cosmic-ray-aerosol-cloud mechanism to explain solar-climate variability (Dickinson 1975). More than 20 years later, correlations between cosmic-ray changes and clouds were reported for the first time by two groups (Svensmark and Friis-Christensen 1997; Pudovkin and Veretenenko 1997). Since these initial observations, a large number of papers have been published that either dispute or support the presence of significant correlations between cosmic rays and clouds, so the atmospheric observations are not yet settled. Carefully controlled laboratory experiments provide the best way of understanding whether or not cosmic rays could affect Earth’s clouds and climate because atmospheric measurements are affected by many uncontrolled sources of variability. This is precisely the aim of the CLOUD experiment at CERN.
In its first round of measurements, the CLOUD experiment is tackling one of the most challenging and long-standing problems in atmospheric science: to understand how new aerosol particles are formed in the atmosphere and the effect that these particles have on climate. Increases in the number concentration of atmospheric aerosol particles cool the climate both directly, by reflecting more sunlight, and indirectly, by forming additional cloud droplets, which makes clouds brighter and extends their lifetimes. The increased amount of aerosols in the atmosphere caused by human activities is thought to have offset a large fraction of the warming caused by greenhouse gases.
By current estimates, about half of all cloud droplets are formed on aerosol particles that were nucleated, so the nucleation process is likely to be important for climate. However, the physical mechanisms of nucleation are not well understood, so aerosol nucleation in current global-climate models is either based on theoretical calculations or adjusted to match observations. The CLOUD collaboration aims to understand the nucleation process and provide reliable aerosol physics for climate models. These data will help to quantify the direct and indirect radiative effects of aerosols on the climate, which are recognized as the largest source of uncertainty in climate forcing contributed by mankind.
To answer these questions, the CLOUD collaboration has built a 3-m stainless-steel chamber with much lower concentrations of contaminants than all previous experiments. This allows the measurement of nucleation from controlled amounts of selected trace gases without the complicating effect of undetected gases. CERN know-how has been key in achieving the demanding technical requirements for the CLOUD chamber and its gas and thermal systems: impurities of condensable vapours in the chamber must be kept below about 1 part per trillion; and the temperature stability of the chamber must be around 0.01 K. CLOUD uses a secondary beam from the CERN Proton Synchrotron (PS) to simulate the effects of cosmic rays with precise control of the “cosmic ray” intensity. The experiment has several other unique aspects, including the capability to create an ion-free environment with an internal electric clearing field, precise control of light-induced (photolytic) gas-phase reactions by means of ultraviolet (UV) illumination from a fibre-optic system, as well as highly stable operation at any temperature between 300 K and 183 K. During experimental runs, small amounts of the chamber atmosphere are extracted and passed through an array of state-of-the-art mass spectrometers and other instruments to measure the ultralow concentrations of atmospheric vapours and other important quantities.
The first results
In its first results published in Nature, the CLOUD collaboration reports on its measurements of the formation of new particles from sulphuric acid, ammonia and water vapours, which have long been thought to account for nucleation in the Earth’s atmosphere. The experiment has also measured the enhancement of atmospheric aerosol nucleation from galactic cosmic rays and the report includes the first-ever measurements of the chemistry and growth, molecule-by-molecule, of newly-formed charged clusters from single molecules up to stable aerosol particles.
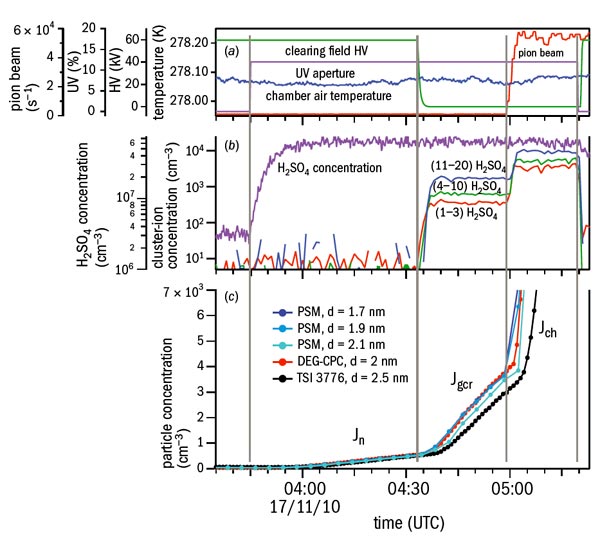
Figure 1 shows a typical sequence of online measurements of the nucleation rates under different ionization conditions. High voltage is initially applied to the clearing-field electrodes to sweep ions from the chamber and suppress all effects of ionization (a). The run is started by opening the shutter of the UV system at a selected aperture, which rapidly establishes a chosen sulphuric-acid concentration in the chamber by photolytic oxidation of SO2 in the presence of O3 and H2O – as occurs in the real atmosphere (b). Particles begin to appear in each aerosol counter after a time delay that depends on the particle growth rate and the detection size threshold (c). When the neutral nucleation rate, Jn, has been measured, the clearing field is turned off (a). This allows cosmic rays to generate ion pairs that remain in the chamber, as shown by the appearance of small ion clusters (b). The ions give rise to a distinct increase in the nucleation rate, Jgcr, resulting from ion-induced nucleation at ground-level cosmic-ray intensity (c). In the next step, a 3.5 GeV/c pion beam from the PS is turned on and passes through the chamber, producing a further sharp increase in the nucleation rate, corresponding to Jch. Finally, the run is ended by closing the UV shutter and turning on the clearing field high-voltage, which starts to clear the chamber of aerosols in preparation for a new run under different conditions.

CLOUD has already made several important discoveries. First, the experiment has shown that the most likely nucleating vapours, sulphuric acid and ammonia, cannot account for the nucleation that is observed in the lower atmosphere. The nucleation measured in the chamber occurs at only 1/10–1/1000 of the rates observed in the lower atmosphere. It is clear from these first results from CLOUD that the treatment of aerosol formation in climate models will need to be revised substantially because all models assume that nucleation in the lower atmosphere is caused by these vapours and water alone. It is now essential to identify the additional nucleating vapours and whether their sources are mainly natural or from human activities. If the vapours have strong anthropogenic sources then there potentially exists a new climate-forcing agent from human activities. Alternatively, if the source is natural, then there is the potential for a new climate feedback that may affect the understanding of how the climate responds to radiative forcings.
Second, CLOUD has found that natural rates of atmospheric ionization caused by galactic cosmic rays substantially enhance nucleation under the conditions studied so far – by up to a factor of 10. Ion-enhancement is particularly pronounced in the cool temperatures of the mid-troposphere (about 5 km altitude) and above. CLOUD has found that at these temperatures, sulphuric acid and water vapour can nucleate without the need for additional vapours. This result leaves open the possibility that cosmic rays could also influence climate. However, it is premature to conclude that cosmic rays have a significant influence on clouds and climate until the additional nucleating vapours have been identified, their ion enhancement measured and the ultimate effects on clouds have been confirmed. So far, CLOUD has only measured the formation rate of aerosols in the few-nanometre size range, which are far too small to seed clouds.
The next steps for the CLOUD experiment will be to investigate the role of biogenic organic vapours in atmospheric aerosol nucleation, to measure condensational growth of aerosols up to sizes sufficient to seed cloud droplets and to study the effect of cosmic rays on these processes. Also, during the next few months, a new fast expansion system will be installed on the CLOUD chamber to allow it to operate as a classical Wilson cloud chamber for the in situ creation of liquid and ice clouds. This will extend CLOUD’s capability to study the effects of cosmic rays directly on cloud droplets and ice particles themselves.
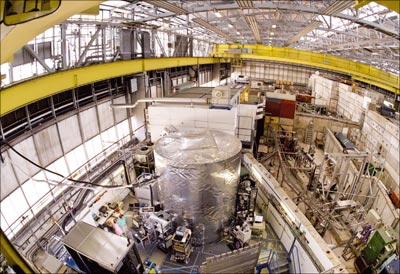
When he visited the Ben Nevis Observatory in 1894 and 1895, Wilson was fascinated by the electrical and cloud condensation phenomena he witnessed. He returned to the Cavendish Laboratory at Cambridge determined to recreate clouds in the laboratory and study their physics. This led to his expansion cloud chamber, later described by Ernest Rutherford as “the most original and wonderful instrument in scientific history”. After his Nobel prize in 1927, Wilson returned to his passion for meteorological phenomena and devoted the rest of his life to the study of atmospheric electricity and clouds. Today, a century after its invention, Wilson’s cloud chamber remains “the most original and wonderful instrument” for studying the link between cosmic rays and clouds.
• The CLOUD (Cosmics Leaving OUtdoor Droplets) experiment is conducted by an international and interdisciplinary collaboration of scientists from Austria (University of Innsbruck, University of Vienna), Finland (Finnish Meteorological Institute, Helsinki Institute of Physics, University of Eastern Finland, University of Helsinki), Germany (Johann Wolfgang Goethe University Frankfurt, Leibniz Institute for Tropospheric Research), Portugal (University of Beira Interior, University of Lisbon), Russia (Lebedev Physical Institute), Switzerland (CERN, Paul Scherrer Institut), the UK (University of Manchester, University of Leeds) and the US (California Institute of Technology). CLOUD has received invaluable support from CERN accelerator and technical teams including, in particular, PH-DT, EN-CV, EN-MME, EN-MEF and TE-VSC.