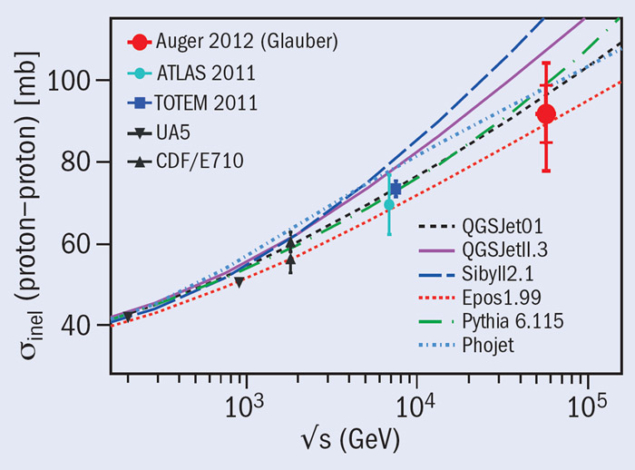
Ultra-high-energy cosmic-ray particles constantly bombard the atmosphere at energies far beyond the reach of the LHC. The Pierre Auger Observatory was constructed with the aim of understanding the nature and characteristics of these particles using precise measurements of cosmic-ray-induced extensive air showers up to the highest energies. These studies allow Auger to measure basic particle interactions, recently in an energy range equivalent to a centre-of-mass energy of √s = 57 TeV.
The structure of an air shower is complex and depends in a critical way on the features of hadronic interactions. Detailed observations of air showers in combination with astrophysical interpretations can provide specific information about particle physics up to √s = 500 TeV. This corresponds to an energy of 1020 eV for a primary proton in the laboratory system.
The depth in the atmosphere at which a cosmic-ray air shower reaches its maximum size, Xmax, correlates with the atmospheric depth at which the primary cosmic-ray particle interacted. The distribution of the measured Xmax values for the most deeply penetrating showers exhibits an exponential tail, the slope of which can be directly related to the interaction length of the initiating particle. This, in turn, provides the inelastic proton–air cross-section. The proton–proton (pp) cross-section is then inferred using an extended Glauber calculation with parameters derived from accelerator measurements that have been extrapolated to cosmic-ray energies. This Auger analysis is an extension of a method first used in the Fly’s Eye experiment in Utah (Baltrusaitis et al. 1984).
The composition of the highest-energy cosmic rays – whether they are protons or heavier nuclei – is not known and the purpose of the Auger analysis is to help in understanding it. The analysis targets the most deeply penetrating particles and so is rather insensitive to the nuclear mix. As long as there are at least some primary protons, then it is their cross-section that is measured. Moreover, to minimize systematic uncertainties, the Pierre Auger collaboration has chosen the cosmic-ray energy range of 1018–1018.4 eV (√sNN ˜ 57 TeV) in which protons appear to constitute a significant contribution to the overall flux. The largest uncertainty arises from a possible helium contamination, which would tend to yield too large a proton inelastic cross-section.
The figure shows the experimental result, which is to be published in Physical Review Letters (Abreu et al. 2012). It confirms the cross-section extrapolations implemented in interaction models that predict a moderate growth of the cross-section beyond LHC energies and is in agreement with the ln2(s) rise of the cross-section expected from the Froissart bound.
Further reading
P Abreu et al. 2012 Phys. Rev. Lett., in press.
R Baltrusaitis et al. Phys. Rev. Lett. 52 1380.