A new kind of X-ray polarimeter giving greatly improved sensitivity and based on methods developed for particle physics gives astronomers a new handle on X-ray measurement.
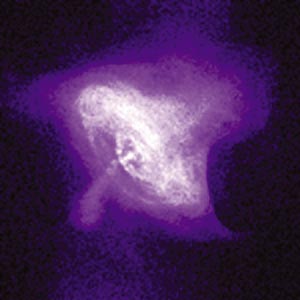
In astronomy there are basically four types of observation that can be made using electromagnetic radiation (photons): measuring the photon direction (imaging), measuring their energy and frequency (spectroscopy), measuring their polarization (polarimetry) and counting the numbers of photons (photometry). These techniques provide complementary information and so are vital for exploring different wavelengths of radiation.
So far, astronomers have not been able to detect efficiently the polarization of photons at X-ray wavelengths, but this should be changed with a new polarimeter for space-borne observations that has been developed in Rome CNR and Pisa INFN by two teams led by Enrico Costa and Ronaldo Bellazzini respectively (Cash 2001).
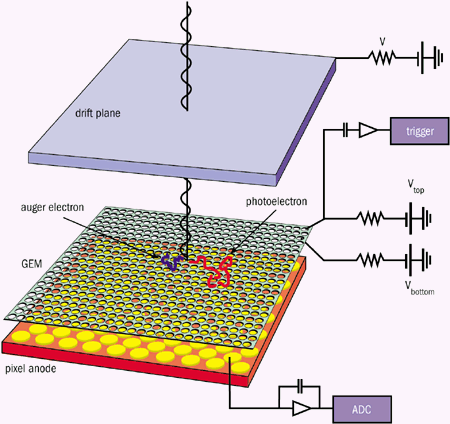
X-ray astronomy has revealed some of the most violent and compact spots in the universe, such as the surfaces of pulsars, close orbits around giant black holes and the blast waves of supernova explosions. The current flagship of X-ray astronomy is NASA’s Chandra observatory (see “http://chandra.harvard.edu”).
By making efficient use of the few photons emitted by discs around black holes and other objects, X-ray astronomers have successfully applied photometry, imaging and spectroscopy to these hot, energetic and often variable sources. Polarimetry has been largely ignored at X-ray wavelengths because of the inefficiency of existing instruments. Yet such a technique could provide a direct picture of the state of matter in extreme magnetic and gravitational fields, and it has the potential to resolve the internal structures of compact sources that would otherwise remain inaccessible. The new X-ray polarimeter, which was developed by the teams in Rome and Pisa, promises to revolutionize space-based observations.
Classic measurements
The first and only generally-accepted measurement of polarized X-rays from an astronomical object dates from more than a quarter of a century ago when a Bragg crystal polarimeter in orbit around the Earth was used to observe the Crab nebula (Weisskopf et al. 1976). This is a remnant of a supernova and is unusual because it has a bright pulsar owing to a neutron star at its core. Spinning at about 30 times per second, the intense magnetic field of this star leaves an indelible imprint on high-energy particles and X-rays.
High-energy electrons that are forced to follow a curved path by a magnetic field emit synchrotron radiation as they change direction. The vibrating electric and magnetic fields of the X-rays are characterized by a polarization angle, which describes the extent to which the fields in the individual photons line up. Synchrotron emission is the source of the polarization detected in the Crab nebula. Being able to measure the polarization as a function of its position across the Crab nebula would reveal a much better picture of the geometry of
the magnetic field in the nebula and its central pulsar.
There has been no unambiguous detection of X-ray polarization from a celestial source since the Crab discovery – all other sources are too faint and/or not sufficiently polarized. To capture the polarization of these faint sources requires a device capable of measuring the polarization angle of every photon collected by an X-ray telescope.
Italian device
The new instrument developed by the Italian teams functions mainly as a photon-counting detector, its overall architecture being similar to a radiation Geiger counter or a proportional counter used in particle physics. The main difference is that traditional position-sensitive X-ray gas detectors typically see only the centroid of the charge cloud produced by the photoelectron, the extent of which is the ultimate limit of the space resolution – a sort of noise to be kept as small as possible. The new concept reverses this approach, trying to resolve the track to measure the interaction point and the prime direction of the photoelectron.
The direction of emission of the photoelectron is a very sensitive indicator of the polarization of the parent photon. The motion of the electron is driven by the direction of the electromagnetic field in the original photon, thereby recording the linear polarization of the X-ray. The polarimeter then has to measure not only the presence of the electron, but also the microscopic path that it has taken.
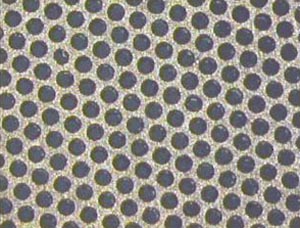
For the first time, photoelectrons of only a few kilo-electronvolts (2-10) are reconstructed not as an indistinct blob of charge but as real tracks. From the detailed study of their momenta it is possible to determine with high efficiency the direction of emission of the photoelectron, which represents the “memory” of the polarization of the incident photon.
When enough events have been detected, the polarization of the emitting source will have been measured. This device makes maximal use of the available information and, when placed at the focus of a large X-ray telescope in orbit, will be able to detect as little as 1% polarization in sources a thousandth of the intensity of the Crab nebula. This will open a new window on the geometry of X-ray-emitting sources.
The instrument used to measure the photoelectron distribution uses micropattern electrode structures of the type developed for particle physics studies. Specifically, the techniques used are the gas electron multiplier (GEM) introduced by Fabio Sauli at CERN in 1996, in which micropores in a thin foil provide a strong field for electron amplification, and a pixel read-out structure using advanced multilayer PCB technology as a collecting anode. The new instrument, being truly two-dimensional (pixel), reveals any polarization direction without having to rotate the detector. The thin GEM foils and the thick film pixel boards used are manufactured at CERN.
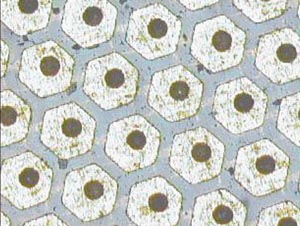
Polarizing mechanisms
Synchrotron radiation is not the only polarizing phenomenon in space; equally important is X-ray scattering. When an X-ray bounces off an electron, as well as losing (or gaining) energy and thereby changing its wavelength, the emerging radiation is also linearly polarized, with its electric field perpendicular to the plane that contains both the incident and the scattered photons. The degree of polarization depends on the angle through which the X-ray scattered, reaching 100% for a deflection of 90°.
This effect can be used to determine the basic geometry of a number of compact X-ray sources.
For a start, the X-ray-emitting regions of active galactic nuclei and quasars demand a closer look. The hot gas swirling into the giant black hole that powers these sources emits copious X-rays through both thermal and non-thermal processes. Narrow jets of particles travelling very close to the speed of light can form by means that are only partially understood. The X-ray spectrum that emerges is complex, featuring emission from several parts of the source (Mushotzky, Done, & Pounds 1993). Radiation passing close to the black hole will be curved and its polarization direction twisted. Furthermore, radiation from one part of the source can scatter off another, creating more features in the spectrum.
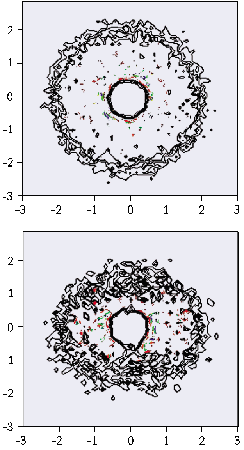
The measurement of polarization as a function of the energy of the X-rays could reveal the history of the scattered radiation and provide a unique test of the physics of these fascinating objects. Significant improvements in observing power usually lead to important new discoveries.
These new precision polarimeters could detect polarization in unexpected places – maybe from the surfaces of thermal neutron stars, or even from interstellar shocks that arise when high-speed plasma collides with quieter regions of cooler gas.
Further reading
W Cash 2001 Nature 411 644-7.
M C Weisskopf et al. 1976 J. Astrophys. 208 L125-L128.
R F Mushotzky, C Done, K A Pounds 1993 Annu. Rev. Astron. Astrophys. 31 717-761.