Founded in 1962, the home of the famous linac went on to make ground-breaking discoveries in particle physics and to evolve in the face of a changing scientific landscape.
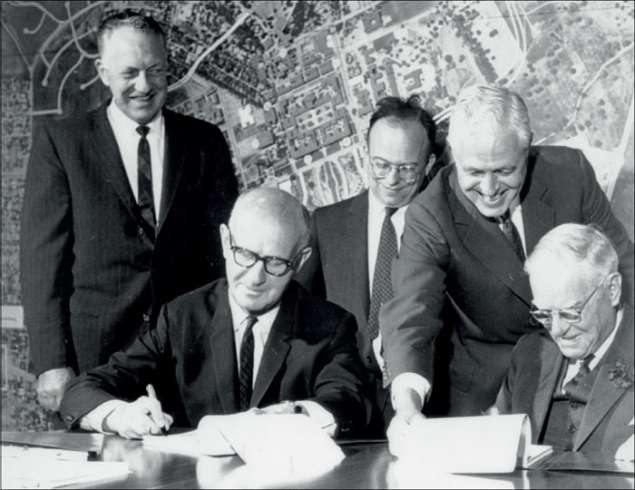
Image credit: Stanford News Service.
In the early 1960s, a 4-km-long strip of land in the rolling hills west of Stanford University was transformed into the longest, straightest structure in the world – a linear particle accelerator. It was first dubbed Project M and affectionately known as “the Monster” by the scientists at the time. Its purpose was to explore the mysterious subatomic realm.
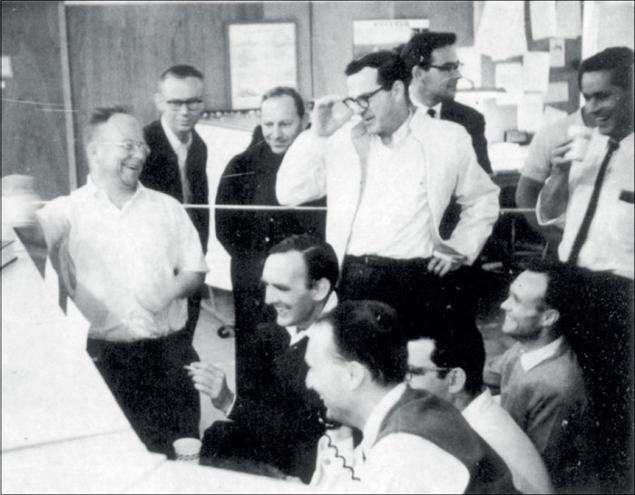
Image credit: SLAC National Accelerator Laboratory.
Fifty years later, more than 1000 people gathered at SLAC National Accelerator Laboratory to celebrate the scientific successes generated by that accelerator and the ones that followed, and the scientists who developed and used them. The two-day event on 24–25 August, for employees, science luminaries and government and university leaders, was more than a tribute to the momentous discoveries and Nobel prizes made possible by the minds and machines at SLAC. It also provided a look ahead at the lab’s continuing evolution and growth into new frontiers of scientific research, which will keep it at the forefront of discovery for decades to come.
A history of discovery
The original linear-accelerator project, approved by Congress in 1961, was a supersized version of a succession of smaller accelerators, dubbed Mark I to Mark IV, which were built and operated at Stanford University and reached energies of up to 730 MeV. The “Monster” would accelerate electrons to much higher energies – ultimately to 50 GeV – for ground-breaking experiments in creating, identifying and studying subatomic particles. Stanford University leased the land to the federal government for the new Stanford Linear Accelerator Center (SLAC) and provided the brainpower for the project. This set the stage for a productive and unique scientific partnership that continues today, supported and overseen by the US Department of Energy.

Image credit: SLAC National Accelerator Laboratory.
Soon after the new accelerator reached full operation, a research team that included physicists from SLAC and Massachusetts Institute of Technology (MIT) used the electron beam in a series of experiments starting in 1967 that provided evidence for hard scattering centres within the proton – in effect, the first direct dynamical evidence for quarks. That research led to the awarding of the 1990 Nobel Prize in Physics to Richard Taylor and Jerome Friedman of SLAC and Henry Kendall of MIT.
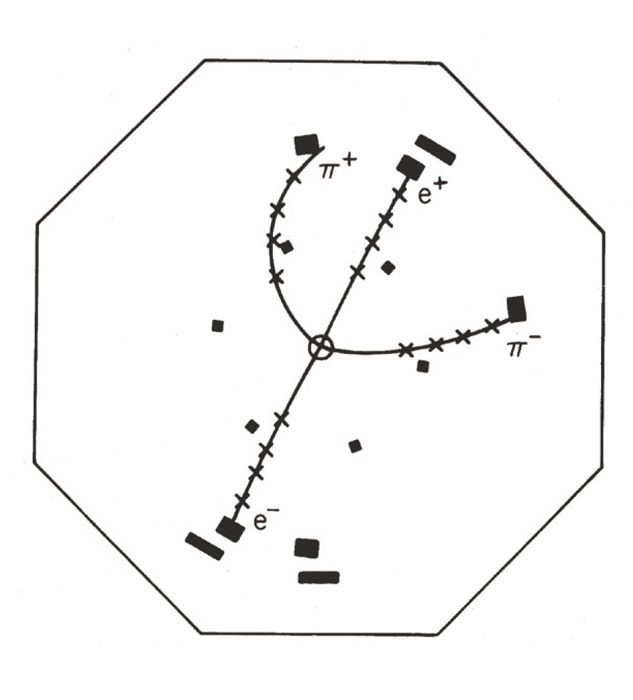
Image credit: SLAC National Accelerator Laboratory.
SLAC soon struck gold again with discoveries that were made possible by another major technical feat – the Stanford Positron Electron Asymmetric Ring, SPEAR. Rather than aiming the electron beam at a fixed target, the SPEAR ring stored beams of electrons and positrons from the linear accelerator and brought them into steady head-on collisions.
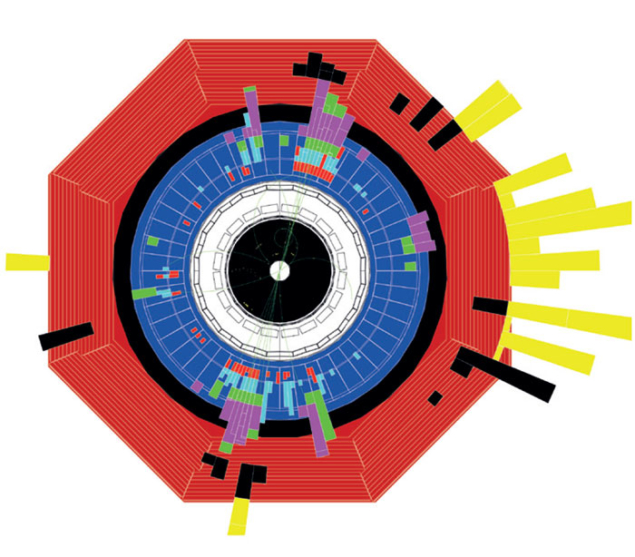
Image credit: SLAC National Accelerator Laboratory.
In 1974, the Mark I detector at SPEAR, run by a collaboration from SLAC and Lawrence Berkeley National Laboratory, found clear signs of a new particle – but so had an experiment on the other side of the US. In what became known as the “November Revolution” in particle physics, Burton Richter at SLAC and Samuel Ting at Brookhaven National Laboratory announced their independent discoveries of the J/ψ particle, which consists of a paired charm quark and anticharm quark. They received the Nobel Prize in Physics for this work in 1976. Only a year after the J/ψ discovery, SLAC physicist Martin Perl announced the discovery of the τ lepton, a heavy relative of the electron and the first of a new family of fundamental building blocks. He went on to share the Nobel Prize in Physics in 1995 for this work.
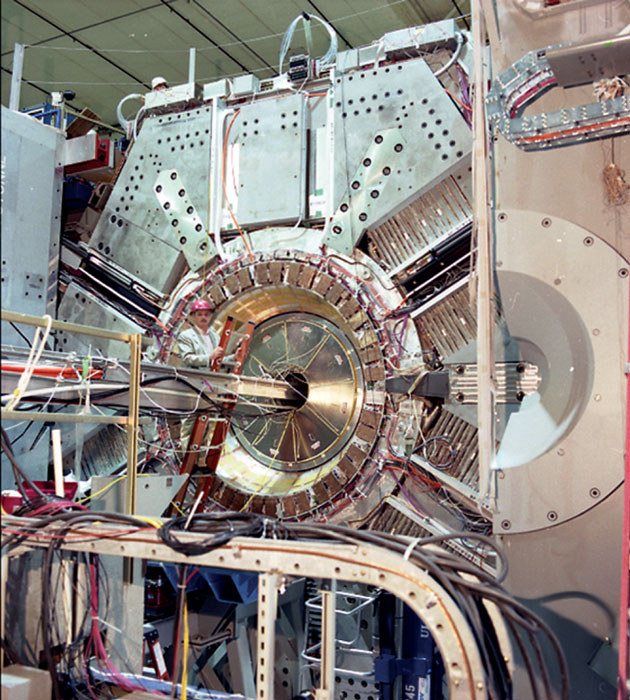
Image credit: SLAC National Accelerator Laboratory.
These and other discoveries that reshaped understanding of matter were empowered by a series of colliders and detectors. The Positron–Electron Project (PEP), a collider ring with a diameter almost 10 times larger than SPEAR, ran during the years 1980–1990. The Stanford Linear Collider (SLC), completed in 1987, focused electron and positron beams from the original linac into micron-sized spots for collisions at a total energy of 100 GeV. Making thousands of Z bosons in its lifetime, the SLC hosted a decade of seminal experiments. It also pioneered the concepts behind the current studies for a linear electron–positron collider to reach energies in the region of 1 TeV.
PEP was followed by the PEP-II project, which included a set of two storage rings and operated in the years 1998–2008. PEP-II featured the BaBar experiment, which created huge numbers of B mesons and their antimatter counterparts. In 2001 and 2004, BaBar researchers and their Japanese colleagues at KEK’s Belle experiment announced evidence supporting the idea that matter and antimatter behave in slightly different ways, confirming theoretical predictions of charge-parity violation.
Synchrotron research and an X-ray laser
Notably, new research areas and projects at SLAC have often evolved as the offspring of the original linear accelerator and storage rings. Researchers at Stanford and SLAC quickly recognized that electromagnetic radiation generated by particles circling in SPEAR, while considered a nuisance to the particle collision experiments, could be extracted from the ring and used for other types of research. They developed this synchrotron radiation – in the form of beams of X-ray and ultraviolet light – as a powerful scientific tool for exploring samples at a molecular scale. This early research blossomed as the Stanford Synchrotron Radiation Project (SSRP), a set of five experimental stations that opened to visiting researchers in 1974.
Its modern descendant, the Stanford Synchrotron Radiation Lightsource (SSRL), now supports 30 experimental stations and about 2000 visiting researchers a year. SPEAR – or more precisely, SPEAR3 following a series of upgrades – became dedicated to SSRL operations 20 years ago. This machine, too, has allowed Nobel-prize winning research. Roger Kornberg, professor of structural biology at Stanford, received the Nobel Prize in Chemistry in 2006 for work detailing how the genetic code in DNA is read and converted into a message that directs protein synthesis. Key aspects of that research were carried out at the SSRL.
Cutting-edge facilities
Meanwhile, sections of the linear accelerator that defined the lab and its mission in its formative years are still driving electron beams today as the high-energy backbone of two cutting-edge facilities: the world’s most powerful X-ray free-electron laser, the Linac Coherent Light Source (LCLS), which began operating in 2009; and FACET, a test bed for next-generation accelerator technologies. LCLS-II, an expansion of the LCLS, should begin construction next year. It will draw electrons from the middle section of the original linear accelerator and use them to generate X-rays for probing matter with high resolution at the atomic scale.
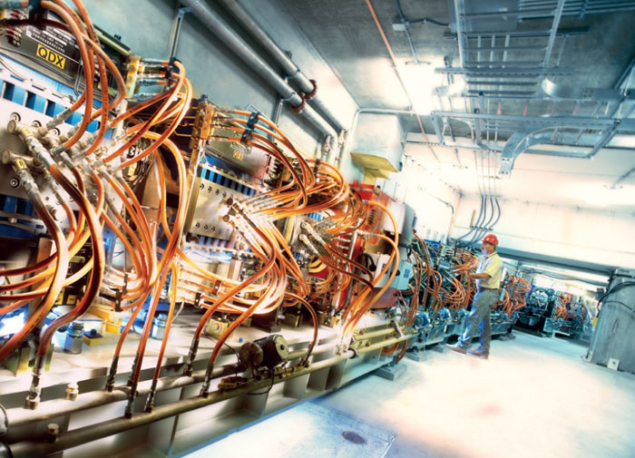
Image credit: SLAC National Accelerator Laboratory.
The late Wolfgang “Pief” Panofsky, who served as the first director of SLAC from 1961 until 1984, often noted that big science is powered by a ready supply of good ideas. He referred to this as the “innovate or die” syndrome. In 1983, Panofsky wrote that he had been asked since the formation of the lab, “How long will SLAC live?” The answer was and still is: “about 10 to 15 years, unless somebody has a good idea. As it turns out, somebody always has had a good idea which was exploited and which has led to a new lease on life for the laboratory.”
Under the leadership of its past two directors – Jonathan Dorfan, who helped launch the BaBar experiment and the astrophysics programme, and Persis Drell, who presided over the opening of the LCLS – SLAC’s scientific mission has grown and diversified. In addition to its original focus on particle physics and accelerator science, SLAC researchers now delve into astrophysics, cosmology, materials and environmental sciences, biology, chemistry and alternative energy research. Visiting scientists still come by the thousands to use lab facilities for an even broader spectrum of research, from drug design and industrial applications to the archaeological analysis of fossils and cultural objects. Much of this diversity in world-class experiments is based on continuing modernizations at the SSRL and the unique capabilities of the LCLS.

Image credit: SLAC National Accelerator Laboratory.
SLAC’s scientists and engineers continue to collaborate actively in international projects – designing machines and building components, running experiments and sharing data with other accelerator laboratories in the US and countries around the globe, including China, France, Germany, Italy, Japan, Korea, Latin America, Russia, Spain and the UK. The lab’s long-standing collaboration with CERN provided an important spark in the formative years of the World Wide Web and led to SLAC’s launch of the first web server in the US. SLAC is also playing an important role in the ATLAS experiment at CERN’s LHC. In the area of synchrotron science, collaborations with US national laboratories and with overseas labs such as DESY in Germany and KEK in Japan have contributed greatly to the development of advanced tools and methodologies, with enormous scientific impact.
Expertise in particle detectors has even elevated the lab’s research into outer space. SLAC managed the development of the Large Area Telescope, the main instrument on board the Fermi Gamma-ray Space Telescope, which was launched into orbit in 2008 and continues to make numerous discoveries. The lab has also earned a role in building the world’s largest digital camera for an Earth-based observatory, the Large Synoptic Survey Telescope, with construction scheduled to begin in 2014 for eventual operation on a mountaintop in Chile.
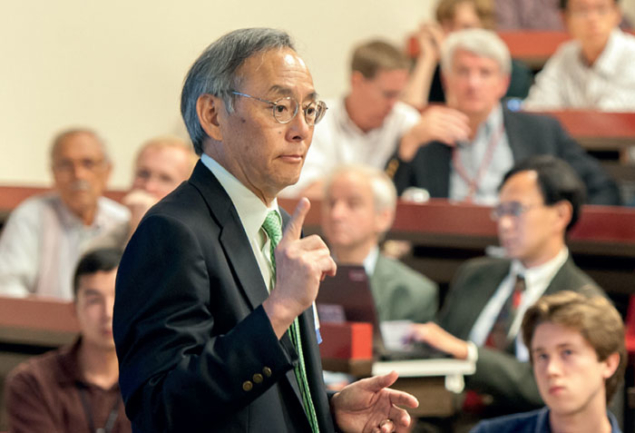
Image credit: SLAC National Accelerator Laboratory.
Richter, who served as SLAC director from 1984 to 1999, has said that the fast-evolving nature of science necessitates a changing path and pace of research. “Labs can remain on the frontiers of science only if they keep up with the evolution of those frontiers,” remarks Richter. “SLAC has evolved over its first 50 years and is still a world leader in areas beyond what was thought of when it was first built. It is up to the scientists of today to keep it moving and keep it on some perhaps newly discovered frontiers for the next 50.”
This article is based on the one published on the SLAC News Centre.
Stanford University, in California, already has a leading position as far as linear accelerators are concerned. It operates a whole family of linacs, several of which are used for medical purposes. The 200 ft machine [Mark III] in operation there produces 700 MeV electrons and its energy will be stepped up to 1050 MeV.
Late in May, Stanford made the scientific headlines – again with a linac.
Addressing a science research symposium in Manhattan, President Eisenhower announced that he would recommend to the US Congress the financing of a “large new electron linear accelerator … a machine two miles long, by far the largest ever built”.
This machine, intended for Stanford University, would be one of the most spectacular atom smashers ever devised. Two parallel tunnels would have to be driven for two miles into the rock of a small mountain in the vicinity of Palo Alto. Such natural cover would, of course, stop any dangerous radiation. One of the tunnels, the smaller in diameter, would house the accelerator proper, while the bigger one would be used for maintenance purposes.
The proposed new linac for Stanford would initially produce 15 BeV (GeV) electrons; it is announced that this energy could later be raised to 40 BeV. It is believed that the machine would take six years to build, at a cost of 100 million dollars. Approval of the project, now only taken after Congressional hearings, depends on the decision to be held in July.
• From the first issue of CERN Courier.