More than just the physics of beauty.
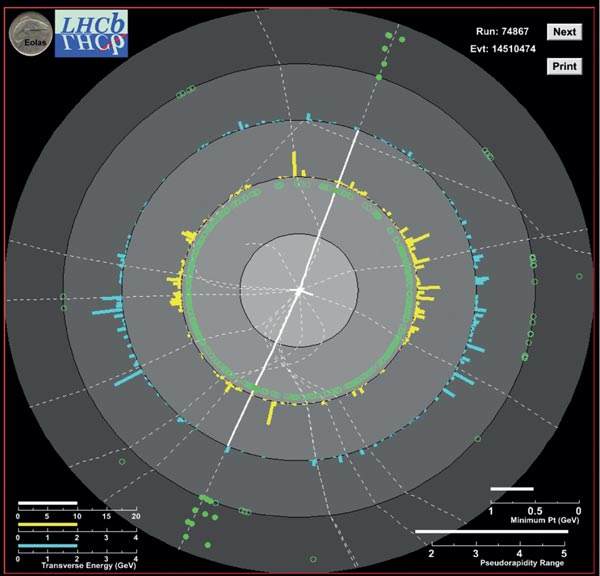
LHCb is one of the four large experiments at the LHC. It was designed primarily to probe beyond the Standard Model by investigating CP violation and searching for the effects of new physics in precision measurements of decays involving heavy quarks, b quarks in particular. At the LHC, pairs of particles (B and B mesons) containing these quarks are mainly produced in the direction of the colliding protons, that is, in the same forward or backward cone about the beam line. For this reason, LHCb was built as a single-arm forward spectrometer that covers production angles close to the beam line with full particle detection and tracking capability – closer even than the general-purpose experiments, ATLAS and CMS. This gives LHCb the opportunity to study the Standard Model in regions that are not easily accessible to ATLAS and CMS. In particular, the experiment has an active and rapidly developing programme of electroweak physics that is beginning to test the Standard Model in several unexplored regions.
Closer to the beam
Particle production at collider experiments is usually described in terms of pseudorapidity, defined as η = –ln(tan θ/2), where θ is the angle that the particle takes relative to the beam axis. The particles tend to be produced in the forward direction: that is crowded into small values of θ, while in terms of η, they are spread more uniformly. The inverse relationship means that the closer a particle is to the beam line, the larger its pseudorapidity. LHCb’s forward spectrometer is fully instrumented in the range 2 < η < 5, a portion of which (2 < η < 2.5) is also covered by ATLAS and CMS. However, the forward region at η > 2.5 – roughly between 10° and 0.5° to the beam – is unique to LHCb, thanks to its full complement of particle detection.
LHCb can explore electroweak physics through the production of W and Z bosons, as well as virtual photons. The experiment can trigger on and reconstruct muons with low momentum pμ > 5 GeV and transverse momentum pTμ > 1 GeV, giving access to low values of the muon-pair invariant mass mμμ > 2.5 GeV. Specialist triggers can even explore invariant masses below 2.5 GeV in environments of low multiplicity. Coupled with the forward geometry, this reconstruction capability opens up a large, previously unmeasured kinematic region.

Figure 1 shows the kinematic regions that LHCb probes in terms of x, the longitudinal fraction of the incoming proton’s momentum that is carried by the interacting parton (quark or gluon), and Q2, the square of the four-momentum exchanged in the hard scatter. Because of the forward geometry, the momenta of the two interacting partons are highly asymmetric in the particle-production processes detected at LHCb. This means that LHCb can simultaneously probe not only a region at high-x that has been explored by other experiments but also a new, unexplored region at small values of x. The high rapidity range and low transverse-momentum trigger thresholds for muons allow potential exploration of Q2 down to 6.25 GeV2 and x down to 10–6, thus extending the region that was accessible at HERA, the electron–proton collider at DESY.
The aim is to probe and constrain the parton-density functions (PDFs) – basically, the probability density for finding a parton with longitudinal momentum fraction x at momentum transfer Q2 – in the available kinematic regions. The PDFs provide important input to theoretical predictions of cross-sections at the LHC and at present they dominate the uncertainties in the theoretical calculations, which now include terms up to next-next-to-leading order (NNLO).
Using data collected in 2010, the LHCb collaboration measured the production cross-sections of W and Z bosons in proton–proton collisions at a centre-of-mass energy of 7 TeV, based on an analysis of about 36 pb–1 of data (LHCb collaboration 2011a). Although only a small fraction of W and Z bosons enter the acceptance of the experiment (typically 10–15%), the large production cross-sections ensure that the statistical error on these measurements is small. The results are consistent with NNLO predictions that use a variety of models for the PDFs. With greater statistics, the measurements will begin to probe differences between these models.
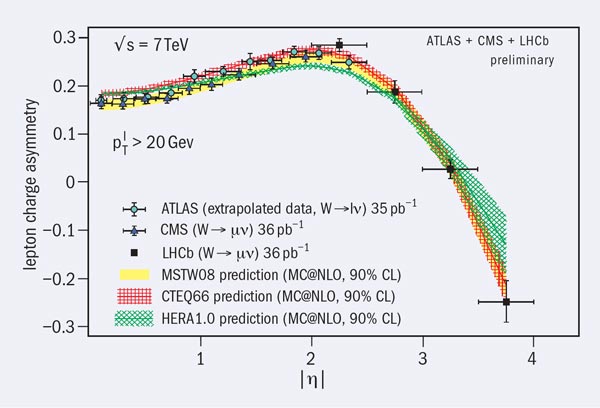
The uncertainty in luminosity dominates the precision to which cross-sections can be determined, so the collaboration also measures ratios of W and Z production, which are insensitive to this uncertainty, as well as the charge asymmetry for W production, AW = ( σW+ – σW– / σW+ + σW–). Figure 2 shows the results for AW overlaid with equivalent measurements by ATLAS and CMS. It illustrates how the kinematic region explored by LHCb is complementary to that of the general-purpose detectors and extends the range that can be tested at the LHC. It is also apparent that LHCb’s acceptance probes the region where the asymmetry is changing rapidly, so the measurements are particularly sensitive to the parameters of the various PDF models.
Low-momentum muons
The LHCb collaboration also plans to increase the probing power of the cross-section measurements by improving the uncertainty in the luminosity itself. Work is ongoing to measure the exclusive production of pairs of muons, a QED process that should ultimately yield a more precise indirect measure of integrated luminosity. Although instrumented in the forward region, LHCb has some tracking coverage in the region –1.5< η< –4 because the proton–proton collision point lies a little way inside the main tracking detector. The measurement exploits this acceptance, LHCb’s ability to trigger on muons with low momentum and the low pile-up environment of collisions at LHCb, which allows the identification of these low multiplicity, exclusively produced events. First measurements based on 2010 data show that the measurement is feasible (LHCb collaboration 2011b). Updated measurements based on the 2011 data set are underway.
In high-energy hadron–hadron scattering the production of Z and W bosons, which decay into lepton pairs, occurs as a Drell-Yan process in which a quark in one hadron interacts with an antiquark in the other hadron to produce a W or a Z or a virtual photon, which then produces a lepton pair of opposite charges. With its ability to trigger on and identify muons with low transverse-momentum, LHCb can measure the production of muon pairs from Drell-Yan production down to invariant masses approaching 5 GeV. As figure 1 shows, these measurements probe values of x around 10–5 and can be used to improve knowledge of the behaviour of gluons inside the proton, building on the knowledge gained at HERA.
These and other production studies are being updated for the upcoming 20th International Workshop on Deep-Inelastic Scattering that takes place in Bonn on 26–30 March. The first measurements using electron final-states will also be available soon, as will those on the production of Z bosons in association with jets. The latter will open the way to more direct probes of the PDFs, once the jets can tagged by flavour (for example, a measurement of the production of a W boson together with a charm jet will allow constraints to be placed on the behaviour of the strange quark inside the proton).
The forward acceptance of LHCb also provides unexpected advantages for other measurements. The further forward in pseudorapidity that final states are produced, the more likely they are to arise from interactions between a valence quark in one proton and an antiquark in the “sea” of the other proton. This is in contrast to the ATLAS and CMS experiments, which experience predominately sea–sea collisions. The measurement of the forwards–backwards asymmetry of Z bosons, which is sensitive to the electroweak mixing angle, sin2θW, benefits from this ability to define a “forward” incoming-quark direction. Studies show that LHCb can identify this correctly in more than 90% of events that have boson rapidities above 3 (McNulty 2011). PDF uncertainties are also reduced in this region. This gives the LHCb experiment the potential to reach the precision of a typical measurement of sin2θW at the Large Electron–Positron collider, even with the data set of 1 fb–1 already recorded.
Studies of the production of the top quark could also benefit from LHCb’s detection system. Although the production rate for top inside LHCb is small at 7 TeV, at 14 TeV the rate should be large enough to make measurements viable. At this centre-of-mass energy, top-pairs are produced by quark–antiquark annihilation twice as often inside the forward region of LHCb’s acceptance as they are in the central region. A measurement of the t-t asymmetry with LHCb could give a direct and comparable cross-check of the recent result from Fermilab’s Tevatron.
Electroweak physics at LHCb may not have been part of the original programme, but the future prospects are bright.