The NUSKY 2011 workshop took place as studies of cosmic neutrinos enter the cubic-kilometre era.
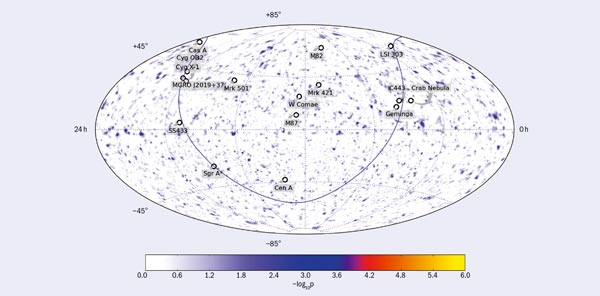
Image credit: Juan Aguilar/IceCube.
Astrophysical neutrinos are produced in the interactions of cosmic rays with an ambient medium of gas (protons) and photons of different energies. Once produced, these cosmic neutrinos can propagate cosmological distances and reach the Earth practically without interactions. They therefore carry unique information about the sources of cosmic rays, their acceleration and the composition of the most energetic phenomena in the universe.
The neutrino sky “seen” by experiments originates in the atmosphere, which shines day and night in neutrinos. One experiment alone, IceCube at the South Pole, has already detected more than 105 atmospheric neutrino events. However, the hope is to see “stars in broad daylight” through this atmospheric flux – that is, to observe neutrinos of cosmic origins. These include neutrinos from various point-like sources and some extended objects, as well as diffuse neutrino fluxes. The selection of the energy band – cosmic neutrinos should dominate at high energies – together with directional and time features, as well as correlations with known objects, emitting for instance in γ rays, are the main tools for distinguishing atmospheric and cosmic neutrinos.
The NUSKY 2011 international workshop on cosmic rays and cosmic neutrinos took place at the Abdus Salam International Centre for Theoretical Physics, Trieste, on 20–24 June. It attracted around 90 participants and featured some 40 talks by the main players in the field, covering all of the important aspects of the production, propagation and detection of high-energy cosmic neutrinos. Numerous discussions ensued, focusing on the implications of the latest experimental results, as well as on the status and perspectives of the field.
The results from IceCube played a prominent role in the discussions
The workshop took place during a critical period for a field in which the working experiments have reached the sensitivity necessary to probe realistic theoretical predictions. The results from IceCube – the first cubic-kilometre-scale detector ever built – thus played a prominent role in the discussions. Their preliminary results correspond to 40 and 59 detector strings (IC40 and IC59); data from IC79 are being analysed and the complete detector, IC86, is now running. So far, the various searches have found no cosmic-neutrino events.
Diffuse neutrino fluxes include the cosmogenic neutrinos generated in cosmic-ray interactions with the photons of the cosmic microwave background, as well as the integrated fluxes from remote, faint and unresolved objects. The IceCube collaboration finds no deviation of the reconstructed neutrino-energy spectrum from that for atmospheric neutrinos. This gives an upper bound on the neutrino flux in the 0.1–10 PeV energy range that is already below the Waxman-Bahcall limit, derived from the known cosmic-ray flux above 1019 eV.
As far as individual sources are concerned, the main suspects are objects that are relatively close, where the acceleration of cosmic rays probably occurs. These include supernova remnants (SNRs) in the Galaxy, as well as active galactic nuclei and gamma-ray bursters (GRBs). The IceCube all-sky maps show no statistically significant signal for steady or transient galactic or extragalactic sources. Nor has any neutrino been detected by IceCube (IC40 + IC59) in the so called stacking analysis of the GRBs (more than 100). The limit on the neutrino flux that emerges from this analysis is a factor of 5 below predictions, thus disfavouring the fireball model of GRBs.
The Pierre Auger Observatory in Argentina and ANITA, the balloon-bourne radio-interferometer that flew over Antarctica, are sensitive to the upper end of the cosmic-neutrino spectrum, the most relevant range for cosmogenic neutrinos (i.e. 1018 eV or 1 EeV). No neutrino-candidate events have been found in Auger data for periods equivalent to two years of the full array. ANITA-II has one candidate event, with one background event expected; cosmogenic models predict from 0.3 to 25 events.
The predictions for atmospheric neutrino fluxes depend on the properties of cosmic rays and on the physical conditions of the sources. In this connection, there are some new and interesting results. IceCube has found cosmic-ray anisotropies in the 20–400 TeV energy range, with a significant angular structure in the southern hemisphere. Anisotropy at higher energies, above 100 TeV, could reveal some connection to nearby SNRs. In addition, the KASCADE-Grande extensive air-shower array has observed structures in the “knee” region of the all-particle cosmic-ray spectrum.
Cosmic-ray origins
Turning to the question of the composition of ultra-high-energy cosmic rays (UHECRs), there had been somewhat contradictory results from the HiRes experiment and the Pierre Auger Observatory. In this connection, the possibilities for UHECR production by sources in the Galaxy (such as past GRBs ), as well as a dominant contribution from Centaurus A, were discussed at the workshop. The basic principles of cosmic-ray acceleration in SNRs are well understood on the basis of the non-linear theory of diffusive acceleration at collisionless Newtonian shocks.
The neutrino−γ-ray connection was at the centre of many discussions as a result of the wealth of new information from γ-ray astronomy. The production of neutrinos should be accompanied by production of γ-rays from π0-decay (the hadronic mechanism). However, ultrahigh-energy γs from extragalactic sources and γs of cosmogenic origin can interact with the medium (photons, electrons), giving rise to electromagnetic cascades. Hence, the whole γ spectrum shifts to lower energies in the giga- to tera-electron-volt range, where the Large Area Telescope (LAT) on the Fermi Gamma Ray Telescope gives important bounds. The Fermi-LAT results on the extragalactic γ flux can be translated into bounds on cosmic rays and cosmogenic neutrinos – the so-called “cascade” bound, based on the approximate equality of the energy released in neutrino production and in the electromagnetic cascade process. These data challenge the GRB origin of cosmic rays: if GRBs are the source of cosmic rays, then 10 events are predicted, while nothing appears in the diffuse bound.
One open question concerns the mechanism for the production of photons at the source. Tera-electron-volt γ-rays from transparent galactic sources can provide a direct indication of cosmic-ray acceleration sites. However, γs can be produced by accelerated electrons via the inverse Compton effect and by synchrotron radiation (both leptonic mechanisms). Fermi-LAT has measured γ spectra from a large number of SNRs and it turns out that both leptonic and hadronic γ-ray models work for SNRs on a source-by-source basis. In the case of GRBs, only bright GRBs are favoured by the Fermi-LAT data as the detectable sources. Nevertheless, bright nearby GRBs seem to be rare.
Features of neutrino propagation are a key element when the flavour of neutrino is taken into account in the detection process. The flavour composition and its dependence on neutrino energy are determined by conditions at the neutrino sources, in particular by the strength of magnetic field, the density distribution, etc. Flavour is also affected by neutrino oscillations and therefore depends on neutrino parameters. The expected composition ratio has the form a : 1 : 1 with a around 1, its precise value depending on 1–3 mixing, the deviation of 2–3 mixing from maximal, the neutrino-mass hierarchy and CP-violation. Various effects typical of physics beyond the Standard Model, such as neutrino decay, non-standard neutrino interactions or the presence of new neutrino species, can also modify the ratio. Finally, the ratio is extremely sensitive to possible violations of fundamental symmetries, such as Lorentz symmetry or the equivalence principle, which lead to modifications in the dispersion relations.
Neutrino astronomy enters a new cubic-kilometre era
Another highlight of the workshop was the report on the first-year of data-taking by DeepCore, the inner detector of IceCube, which has a low energy threshold of 10 GeV. The rate of events, which include cascades induced by electron-neutrinos as well as neutral current muon-neutrinos, was shown. DeepCore will detect around 800 neutrino-induced cascades per year. The physics motivations for the Phased IceCube Next Generation Upgrade (PINGU-I and PINGU-II) were also presented.
Neutrino observatories have now reached sufficient sensitivity to constrain multimessenger signals, γ-rays and UHECRs with minimal assumptions. That there is no evidence as yet for astrophysical neutrinos poses a problem for future projects because it means that IceCube will only scratch the surface of neutrino astronomy. The prime targets now are the transient sources.
There are several projects already under consideration or in progress. KM3NeT, a detector for neutrino astronomy under the Mediterranean Sea, which will have an instrumented volume of more than 5 km3, is in its preparatory phase. It will search for neutrino point sources in the energy range 100 GeV – 1 PeV. The Cherenkov Telescope Array is a new instrument for very high-energy (10–105) GeV γ astronomy. JEM-EUSO will detect Cherenkov light coming from the atmosphere using a telescope on the International Space Station that will have an instantaneous aperture of up to 106 km2. ANITA-III, approved to fly in 2013–2014, will search for ultra-high-energy neutrinos with 3–5 times higher sensitivity than ANITA-II. The Askaryan Radio Array is a ground-based antenna array at the South Pole covering an area of 100 km2. The expected yield is 3–5 neutrinos per year above 1017 eV, below the bulk of the cosmogenic neutrino predictions.
The NUSKY 2011 workshop was held just as high-energy neutrino astronomy enters a new cubic-kilometre era. Current bounds already have important implications and any further improvement of data will have an impact on the picture of the neutrino sky, with important consequences. The hope is that, with progressively more data from IceCube, a discovery is on the horizon. As Francis Halzen, of the University of Wisconsin-Madison and IceCube, concluded, “Hess 1912… and still no conclusion [on the origins of cosmic rays]; now the instrumentation is in place… SNRs and GRBs are in close range!”