The largest liquid-argon time-projection chamber is now in action.

Historically, imaging detectors have played a crucial role in particle physics. In particular, bubble-chamber detectors – such as Gargamelle at CERN – were an incredibly fruitful tool, permitting the visualization and measurement of particle interactions in an unprecedented way and providing fundamental contributions, in particular in neutrino physics. However, in the search for rare phenomena, bubble chambers are limited mainly by the impossibility to scale their size to larger masses and by their duty cycle, which is intrinsically limited by the mechanics of the expansion system.
The concept of the liquid-argon time-projection chamber (LAr-TPC) was conceived more than 30 years ago: it allows the calorimetric measurement of particle energy together with 3D track reconstruction from the electrons drifting in an electric field in sufficiently pure liquid argon (Rubbia 1977). The LAr-TPC successfully reproduces not only the imaging features of the bubble chamber – its medium and spatial resolution being similar to those of heavy-liquid bubble chambers – but it also has the further achievement of being a fully electronic detector, which is potentially scalable to multikilotonne masses. In addition, it provides excellent calorimetric measurements, with the big advantage of being continuously sensitive and self-triggering.
The ICARUS LAr-TPC
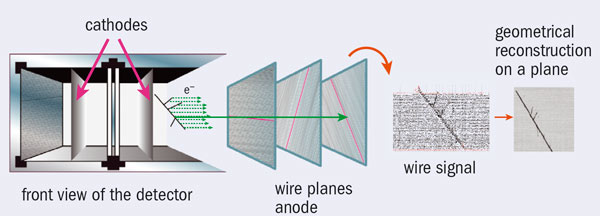
The ICARUS T600, the largest LAr-TPC ever built, contains 760 tonnes of liquid argon (LAr). It represents the state of the art of this technique and marks a major milestone in the practical realization of large-scale LAr detectors. Installed in Hall B of the underground Gran Sasso National Laboratory (LNGS) of the Instituto Nazionale di Fisica Nucleare (INFN), it is collecting neutrino events from the beam of the CERN Neutrinos to Gran Sasso (CNGS) project. Produced at CERN, the neutrinos reach Gran Sasso after a journey of around 730 km. The detector also acts as an underground observatory for atmospheric, solar and supernovae neutrinos. In addition it will search for proton decay (in particular into exotic channels) in one of its 3 × 1032 nucleons, with zero background.
The ICARUS T600 detector consists of a large cryostat that is split into two identical, adjacent half-modules (with internal dimensions of 3.6 × 3.9 × 19.6 m3), which are filled with ultrapure liquid argon (Amoruso et al. 2004). Each half-module houses two TPCs separated by a common cathode, with a drift length of 1.5 m. Ionization electrons, produced by charged particles along their paths, are drifted under a uniform electric field (ED = 500 V/cm) towards the TPC anode made of three parallel wire planes that face the drift volume (figure 1). A total of approximately 54,000 wires are deployed with 3 mm pitch, orientated on each plane at a different angle (0°, +60° and –60°) with respect to the horizontal direction. By appropriate voltage biasing, the first two planes (the induction-1 and induction-2 planes) provide signals in a non-destructive way; finally, the ionization charge is collected and measured on the last plane (the collection plane).
The relative time of each ionization signal, combined with the electron drift-velocity information (vD ˜ 1.6 mm/μs), provides the position of the track along the drift coordinate. Combining the wire coordinate on each plane at a given drift time, a 3D image of the ionizing event can be reconstructed with a remarkable resolution of about 1 mm3. The absolute time of the ionizing event is provided by the prompt UV-scintillation light emitted in the LAr and measured through arrays of photomultiplier tubes (PMTs), installed in the LAr behind the wire planes.

The electronics for data acquisition allow continuous read-out, digitization and independent waveform recording of signals from each wire of the TPCs. The electronic noise is 1500 electrons r.m.s. to be compared with around 15,000 free electrons produced by a minimum-ionizing particle in 3 mm.
To permit electrons produced by ionizing particles to travel “unperturbed” from the point of production to the wire planes, electronegative impurities (mainly O2, H2O and CO2) in the LAr must be kept at a low concentration level (below 0.1 ppb). Therefore, both gaseous and liquid argon are continuously purified by recirculation through standard Hydrosorb/Oxysorb filters.
Preassembly of the ICARUS T600 detector began in 1999 in Pavia and one of the two 300-tonne half-modules was brought into operation in 2001 and tested with cosmic rays at the Earth’s surface. To meet safety and reliability requirements for underground operation in Hall B at LNGS, the ICARUS T600 module – illustrated in figure 2 – was equipped with dedicated technical infrastructures. Assembly of the complete detector was achieved in the first months of 2010 and it was finally brought into operation with its subsequent commissioning.
Operation at LNGS
In the spring of 2010, the detector was filled with ultrapure LAr and activated immediately. Events from the CNGS neutrino beam and cosmic rays were observed with a trigger system that relied on both the scintillation light signals provided by the internal PMTs and the CNGS proton-extraction time. The “early warning” signal, sent from CERN to LNGS some 80 ms before the first proton spill extraction, allows the opening of two gates of around 50 μs, corresponding to the predicted extraction times. The first observed CNGS neutrino event is shown in figure 3 other beautiful events with a muon crossing both chambers of a module and two neutral pions are shown in the middle and bottom parts of figure 3, respectively.
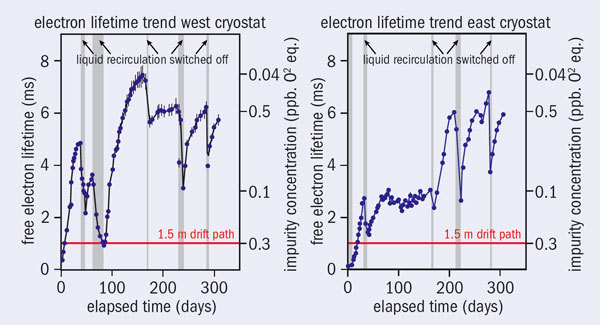
LAr purity is monitored continuously by measuring the charge attenuation along the tracks of ionizing cosmic muons that cross the full drift path. With the liquid recirculation turned on, the LAr purity steadily increased, the value of the free-electron lifetime exceeding 6 ms in both half-modules after a few months of operation (figure 4). This corresponds to a maximum free-electron yield attenuation of 16%. Sudden degradations of purity owing to periodic pump stops for maintenance are always recovered promptly within a few days.
The performance of LAr-TPCs has been studied progressively over the past two decades by exposing different detectors to cosmic rays and neutrino beams, culminating in the successful achievement of the T600 operation. The high resolution and granularity of the detector imaging allow the precise reconstruction of event topology, which is completed by a calorimetric measurement.
Particles are identified by studying both the dE/dx versus range and the decay/interaction topology. Electrons are identified by the characteristic electromagnetic showering, being well separated from π0 via γγ reconstruction, dE/dx signal comparison and the π0 invariant mass measurement at the level of 10–3. This feature guarantees a powerful identification of the charged current (CC) electron-neutrino interactions, while rejecting neutral-current (NC) interactions to a negligible level. The electromagnetic energy resolution σ(E)/E = 0.03/√(E(GeV)) ⊕ 0.01 is estimated in agreement with the π0 → γγ invariant mass measurements in the sub-giga-electron-volt energy range, while σ(E)/E = 0.30/√(E(GeV)) has been inferred for hadronic showers.

For long muon tracks that escape the detector, momentum is determined by measuring the displacements arising from multiple scattering along the track. The procedure, implemented through a Kalman filter algorithm and validated on stopping muons, allows a resolution of Δp/p that can be as good as 10%.
During the 2010 CNGS run, the T600 acquired neutrino interaction events with steadily increasing efficiency, a live time of up to 90% and increasing quality. In the last 2010 period, about 100 neutrino CC events were collected and classified, in agreement with expectations.
As an example of the detector capabilities, figure 5 shows a CNGS νμ CC event with a 13 m-long muon track, together with zoomed projections on the collection and induction-2 planes. The use of two different views allows the recognition of two distinct electromagnetic showers pointing to – but detached from – the primary vertex. Even though the two photons overlap in the collection view it was possible to determine the associated invariant mass m12* = 125±15 MeV/c2, which is compatible with the π0 mass. The initial ionization of the closer photon amounts to 2.2 minimum ionizing particles. This is a clear signature for pair conversion, thus confirming the expected e/π0 identification capabilities of the detector.

Image credit: Francesco Arnedo LNGS-INFN.
The momentum of the long muon track in figure 5 has been measured to be via the multiple-scattering method pμ = 10.5±1.1 GeV/c. The other primary long track is identified as a pion that interacts to give a secondary vertex. A short track from the secondary vertex is identified as a kaon, decaying in flight into a muon. From the decay topology and energy deposition, the kaon momentum can be evaluated as 672±44 MeV/c.
The capability for identifying and reconstructing low-energy kaons is a major advantage of the LAr-TPC technique for proton-decay searches. In the event described, the kaon momentum is not far from the average (300 MeV/c), for instance in the p → ν– K+ channel. Also, the ability to identify π0s, as in this event, is effective for many nucleon-decay channels, as well as for the discrimination of NC events when looking for νμ → νe oscillations.
The missing transverse-momentum reconstructed is 250 MeV/c. Despite the non-full containment of the event, this value is consistent with the theoretical expectation from the Fermi motion of target nucleons. The reconstructed total energy is 12.6±1.2 GeV, well within the energy range of the CNGS beam (Bailey et al. 1999).