With its new cooling system in place, the Alpha Magnetic Spectrometer continues its quest to understand the origin of cosmic rays.
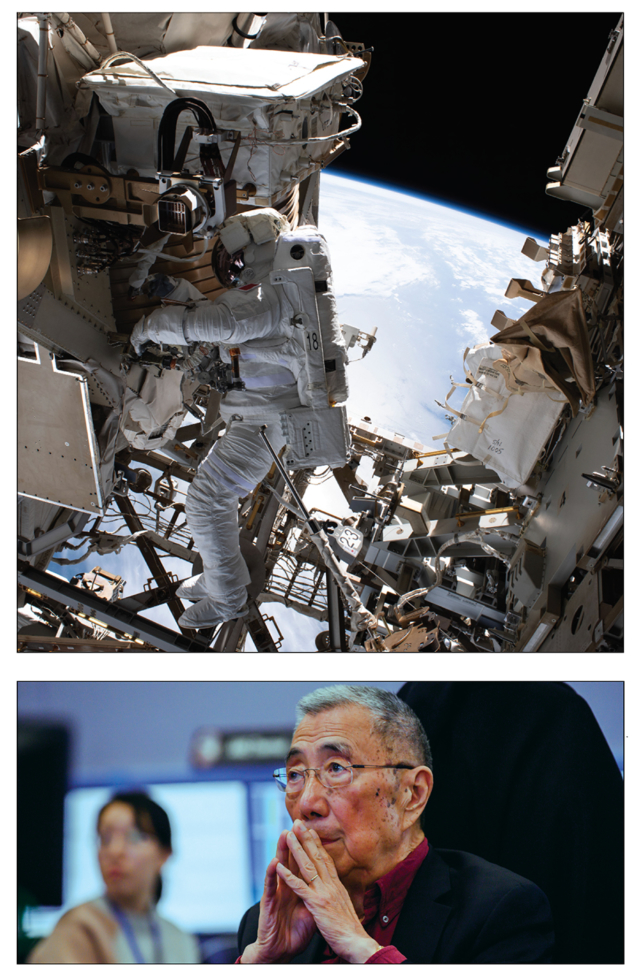
On 25 January, European Space Agency astronaut Luca Parmitano stepped outside a half-million-kilogramme structure travelling at tens of thousands of kilometres per hour, hundreds of kilometres above Earth, and, tethered by a thin cord, ventured into the vacuum of space to check for a leak.
It was the fourth such extravehicular activity (EVA) he’d been on in two months. All things considered, the task ahead was relatively straightforward: to make sure that a newly installed cooling system for the Alpha Magnetic Spectrometer (AMS), the cosmic-ray detector that has been attached to the International Space Station (ISS) since 2011, had been properly plumbed in.
Heart-stopping spacewalks
The first EVA on 15 November saw Parmitano and fellow astronaut, NASA’s Drew Morgan, remove and jettison the AMS debris shield, which is currently still spiralling its way to Earth, to allow access to the experiment’s cooling system. The CO2 pumps, needed to keep the 200,000-channel tracker electronics at a temperature of 10 ± 3 °C, had started to fail in 2014 – which was no surprise, as AMS was initially only supposed to operate for three years. During the second EVA on 22 November, the astronauts cut through the cooling system’s eight stainless-steel lines to isolate and prepare it for removal, and a critical EVA3 on 2 December saw Morgan and Parmitano successfully connect the new pump system, which had been transported to the ISS by an Antares rocket the previous month. Then came a long wait until January to find out if the repair had been successful.
“EVA4 was the heart-stopping EVA because that’s where we did the leak tests on all those tubes,” says Ken Bollweg, NASA’s AMS project manager. The success of the previous EVAs suggested that the connections were going to be fine. But Parmitano arrived at the first tube, attached one of 29 bespoke tools developed specially for the AMS repair, and saw that the instrument had issued a warning signal. “I see red,” he reported to anxious teams at NASA’s Johnson Space Center’s Mission Control Center and the AMS Payload Operations Control Centre (POCC) at CERN’s Prévessin site, from where spokesperson Sam Ting and his colleagues were monitoring proceedings closely. Though not huge, the leak was serious enough not to guarantee that the system would work, jeopardising four years of preparation involving hundreds of astronauts, engineers and scientists. Following procedures put in place to deal with such a situation, Parmitano tightened the connection and waited for about an hour before checking the tube again. A leak was still present. Then, after re-tightening the troublesome connection again, while the team was preparing a risky “jumper” manoeuvre to bypass the leak and make a new connection, he checked a third time: “No red!” Happy faces lit up the POCC.
NASA has learned a lot of new things from this
Ken Bollweg
AMS was never designed to be serviceable, and the repair, unprecedented in complexity for a space intervention, required the avoidance of sharp edges and other hazards in order to bring it back to full operational capacity. The chances of something going wrong were high, says Bollweg. “NASA has learned a lot of new things from this. We really pushed the envelope. It showed that we have the capabilities to do even more than we have done in the past.” EVA4 lasted almost six hours. Five hours and two minutes into it, Parmitano, who returned safely to Earth on 6 February, broke the European record for the most time spent spacewalking (33 hours and nine minutes). It’s not a job for the fainthearted. During a spacewalk in 2013, while wedged into a confined space outside the ISS, a malfunction in Parmitano’s spacesuit caused his helmet to start filling with water and he almost drowned.
“Building and operating AMS in space has been an incredible journey through engineering and physics, but today it is thanks to the NASA group that in AMS we can continue this journey and this is amazing. An enormous thanks to the EVA crew,” said AMS integration engineer Corrado Gargiulo of CERN. The day after EVA4, the POCC team spent about 10 hours refilling the new AMS cooling system with 1.3 kg of CO2 and started to power up the detector. At noon on 27 January, all the detector’s subsystems were sending data back, marking a new chapter for AMS that will see it operate for the lifetime of the ISS.
Into the unknown
The 7.5 tonne AMS apparatus has so far recorded almost 150 billion charged cosmic rays with energies up to the multi-TeV range, and its percent-level results show clear and unexpected behaviour of cosmic-ray events at high energies. A further 10 years of operation will allow AMS to make conclusive statements on the origin of these unexpected observations, says Ting. “NASA is to be congratulated on seeing this difficult project through over a period of many years. AMS has observed unique features in cosmic-ray spectra that defy traditional explanations. We’re entering into a region where nobody has been before.”
AMS has observed unique features in cosmic-ray spectra that defy traditional explanations
Sam Ting
The first major result from AMS came in 2013, when measurements of the cosmic positron fraction (the ratio of the positron flux to the flux of electrons and positrons) up to an energy of 350 GeV showed that the spectrum fits well to dark-matter models. The following year, AMS published the positron and electron fluxes, which showed that neither can be fitted with the single-power-law assumption underpinning the traditional understanding of cosmic rays. The collaboration has continued to find previously unobserved features in the measured fluxes and flux ratio of electrons and positrons, publishing the results in several high-profile papers during the past couple of years.

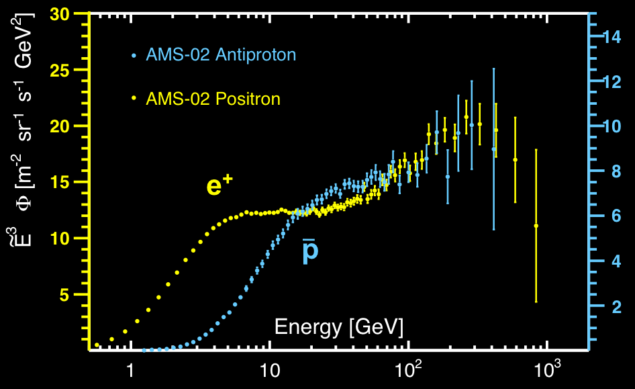
Last year, AMS reaffirmed the complex energy dependence exhibited by the positron flux: a significant excess starting from 25 GeV, a sharp drop-off above 284 GeV and a finite energy cutoff at 810 GeV (figure 1). “In the entire energy range the positron flux is well described by the sum of a term associated with the positrons produced in the collision of cosmic rays, which dominates at low energies, and a new source term of positrons, which dominates at high energies,” says Ting. “These experimental data on cosmic-ray positrons show that, at high energies, they predominantly originate either from dark-matter annihilation or from other astrophysical sources.” Although dark-matter models predict such a cut off, the AMS data cannot yet rule out astrophysical sources, in particular pulsars. Further intrigue comes from the latest, to-be-published, AMS result on antiprotons, which, although rare at high energies, exhibit similar functional behaviour as the positron spectrum (figure 2). “This indicates that the excess of positrons may not come from pulsars due to the similarity of the two spectra and the high mass of antiprotons,” says Ting.
Thanks to the successful installation of the new AMS cooling system, the expected positron spectrum by 2028, in particular the high-energy data points, should enable an accurate comparison with dark-matter models (figure 3). High-energy (>TeV) events are also expected to provide insights into the origins of cosmic electrons, the latest results on which show that the electron flux exhibits a significant excess starting from 42 GeV.

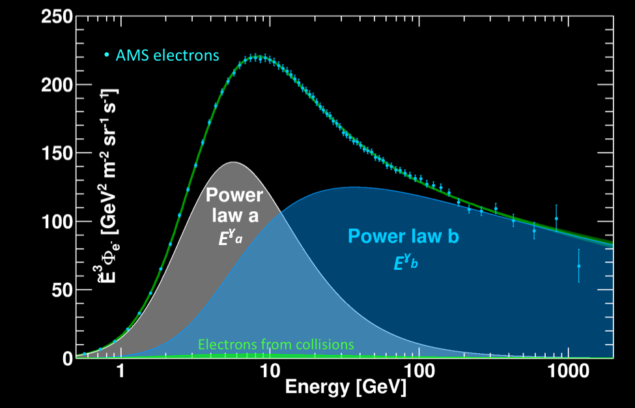
Unlike the positron flux, which has an exponential energy cutoff at 810 GeV, the electron flux does not have a cutoff (at least not below 1.9 TeV). Also: in the entire energy range the electron flux is well described by the sum of two power law components (figure 4), providing “clear evidence”, says Ting, that most high energy electrons originate from different sources than high energy positrons.
Novelties in nuclei
Unexpected results continue to appear in data from cosmic nuclei, which make up the bulk of cosmic rays travelling through space. Helium, carbon and oxygen nuclei are thought to be mainly produced and accelerated in astrophysical sources and are known as primary cosmic rays, while lithium, beryllium and boron nuclei are produced by the collision of heavier nuclei with nuclei of the interstellar matter and are known as secondary cosmic rays.
New properties of primary cosmic rays – helium, carbon and oxygen – have been observed in the rigidity range 2 GV to 3 TV; at high energies these three spectra also have identical rigidity dependence, all deviating from a single power law above 200 GV. Similar oddities have appeared in measurements of secondary cosmic rays – lithium, beryllium and boron – in the range 1.9 GV to 3.3 TV (figure 5). The lithium and boron fluxes have an identical rigidity dependence above 7 GV, all three fluxes have an identical rigidity dependence above 30 GV, and, unexpectedly, above 30 GV the Li/Be flux ratio is approximately equal to two.
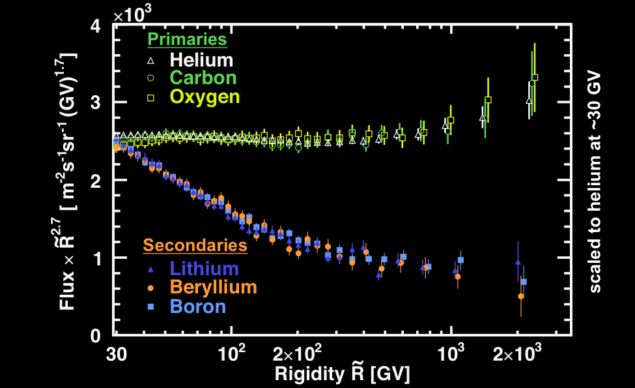
The ratio of secondary fluxes to primary fluxes is particularly interesting because it directly measures the amount and properties of the interstellar medium. Before AMS, only the B/C ratio was measured and was assumed to be proportional to RΔ with Δ a constant for R > 60 GV. The latest AMS results on secondary (Li, Be, B) to primary (C, O) flux ratios show that Δ is not a constant, but changes by more than 5σ between the two rigidity ranges, 60 < R < 200 GV and 200 < R < 3300 GV. As with electron and positron fluxes, none of the current AMS results can be explained by existing theoretical models. By 2028, says Ting, AMS will extend its measurements of cosmic nuclei up to Z=30 (zinc) with sufficient statistics to get to the bottom of these and other mysteries. “We have measured many particles, electrons, positrons, antiprotons and many nuclei, and they all have distributions and none agree with current theoretical models. So we will begin to create a new field.”
Further reading
AMS Collab. 2019 Phys. Rev. Lett. 122 041102.
AMS Collab. 2018 Phys. Rev. Lett. 120 021101.
AMS Collab. 2018 Phys. Rev. Lett. 122 101101.
AMS Collab. 2017 Phys. Rev. Lett. 119 251101.