After three years of construction, the OPERA experiment has detected the first neutrinos from CNGS, and an automated production and assembly line is in full swing to complete the detector so that its full search for the appearance of tau neutrinos can begin.
Neutrino physics is a special field in which large-mass targets, which are needed to detect these elusive particles, are often combined with the ambitious goal of precision measurements, which are needed for firm conclusions. The Oscillation Project with Emulsion-tRacking Apparatus (OPERA) is a good example. It aims to directly observe the appearance of tau neutrinos (ντ) in oscillations from muon neutrinos (νμ→ντ) in the long-baseline beam of the CERN Neutrinos to Gran Sasso (CNGS) project. This would confirm the oscillation hypothesis for atmospheric neutrinos and unambiguously clarify its nature.
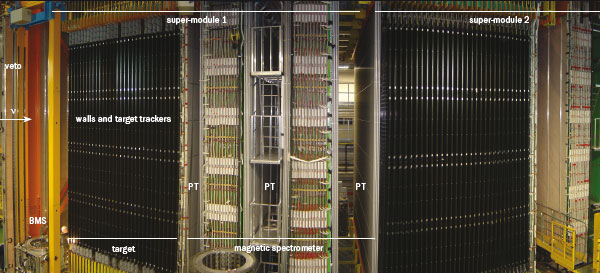
In 1998, the Super-Kamiokande collaboration announced that muon neutrinos change flavour (oscillate). The evidence came from detecting fewer muon neutrinos than expected in showers created by cosmic-ray interactions in the atmosphere. More recently, the KEK-to-Kamioka (K2K) experiment, using a man-made neutrino beam from KEK to the Super-Kamiokande detector, and the MINOS experiment, which uses a neutrino beam from Fermilab, have confirmed the oscillations that were observed in the atmospheric neutrinos. These experiments showed that muon neutrinos disappear, but there is no evidence of what they become. Only detecting the appearance of tau neutrinos from muon neutrinos will confirm the current theories underlying neutrino oscillation.
OPERA is searching for ντ by directly detecting the decay of the tau lepton that is produced in charged-current interactions of the ντ with nucleons in matter. To be sensitive to the oscillation parameters that are indicated by the deficit of muon neutrinos in the Super-Kamiokande data (Δm2 = 1.9–3.1 × 10-3 eV2, 90% CL, full mixing) and confirmed by the K2K and MINOS experiments, the experiment uses a 732 km baseline from the neutrino source to the detector – the distance from CERN to the Gran Sasso National Laboratory (LNGS) under the Gran Sasso massif in Italy. The νμ beam of CNGS has been optimized to obtain the maximum number of ντ charged-current interactions at OPERA and has an average νμ energy of about 17 GeV.
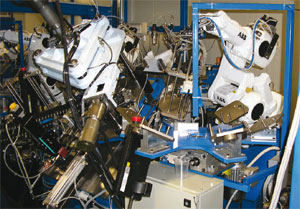
Detecting the ντ through the charged-current interaction is a challenging task. It demands not only a massive neutrino target (about 1.8 kilotonne), but also particle tracking at micrometre resolution to reconstruct the topology of the tau decay: either the kink – a sharp change (> 20 mrad) in direction occurring after about 1 mm – as the original tau lepton decays into a charged particle and one or more neutrinos, or the vertex for the decay mode into three charged particles plus a neutrino. For this purpose, the emulsion cloud chamber (ECC) – exploited by the DONUT collaboration at Fermilab for ντ detection in a beam-dump experiment in 2000 – combines in a sandwich-like cell the high-precision tracking capabilities of nuclear emulsions (two 40 μm layers on both sides of a 200 μm plastic base) and the large target mass provided by lead plates (1 mm thick).
Anatomy of the detector
The design of OPERA is completely modular and allows real-time analysis of neutrino interactions. The target will eventually consist of 206,336 bricks, each comprising 56 consecutive ECC cells with transverse dimensions of 10.2 × 12.7 cm and weighing 8.3 kg. The bricks are being built underground at LNGS in the automated brick-assembly machine (BAM). They are then inserted into the experiment by two robots – the brick manipulator system (BMS) – into planar structures, or walls, which are interleaved with planes of scintillator tracker (5900 m2), built from vertical and horizontal strips of plastic scintillator 2.6 cm wide. The scintillators provide an electronic trigger for neutrino interactions, localize the particular brick in which the neutrino has interacted, and perform a first tracking of muons within the target. Localizing the brick is the first step in locating events in OPERA’s emulsions; the electronic trackers then provide predictions for the search for the particle tracks in the vertex brick with a position resolution of the order of 1 cm and an angular accuracy (on the muon tracks) of about 20 mrad.
The target sections are arranged in two independent super-modules, each with 31 walls and 64 layers of 52 bricks for each wall. Each super-module includes a muon spectrometer after the target section. The study of the muonic tau-decay channel needs muon identification and charge measurement. More generally, they are fundamental handles for suppressing the background from the decay of charmed particles, which are produced in ordinary_νμ charged-current interactions and decay similarly to the tau. The identification, with more than 95% efficiency, of the primary muon from the neutrino interaction that produces the charmed particle, allows this background to be effectively killed.
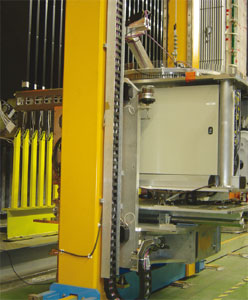
Each muon spectrometer comprises a dipolar magnet made of two iron arms (1 kilotonne of iron magnetized at 1.55 T), interleaved by pairs of 7 m long vertical drift-tube stations, 8064 tubes in total. These are the precision trackers (PTs) for precisely measuring the bending in the spectrometer. Twenty-two planes of resistive plate counters, 1525 m2 for each magnet, are inserted between the iron plates to provide coarser tracking in the magnet and a range measurement of stopping particles. An Ethernet-based data-acquisition system time-stamps and records asynchronously all detector hits in the target trackers and the spectrometers.
Selected bricks can be extracted daily from the target by the BMS for emulsion development and analysis. Each brick is equipped externally with a pair of emulsion sheets – the changeable sheets (CSs) – attached to its downstream face. Once a brick with an event has been localized, the CSs provide a first confirmation of the neutrino interaction and the initialization for the scanning analysis of the brick. Automatic fast microscopes scan large areas of emulsion and perform track reconstruction, searching for the tau-decay topologies and measuring the event kinematics. Track momenta are measured from their multiple scattering in the brick, while energies of electrons and photons are reconstructed from the development of electromagnetic showers.
Industrial production
When OPERA is fully operational, about 30 bricks a day will be extracted from the target with 1800 emulsion foils. The total emulsion surface to be measured corresponds to a few thousand square metres in five years. To cope with the real-time analysis of the neutrino interactions, high-speed automatic scanning microscopes were developed in Europe and Japan. These scan around 20 cm2 an hour – 20 times faster than in previous experiments.
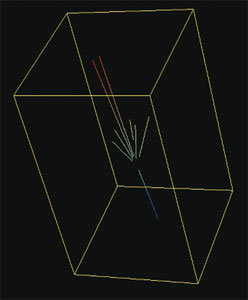
As well as the special development of the microscopes, a large automation effort had to be put in to the brick production and handling, emulsion processing and chemical development, which together represent a small industry. Brick production has to be done underground to minimize the number of background tracks from cosmic rays and environmental radiation – LNGS has an average overburden of 1400 m of rock. Owing to space constraints the bricks must then be inserted immediately into the detector.
BAM specifications imply the production of about 1000 bricks a day, with 0.1 mm accuracy in each dimension. The BAM consists of an automated production line with several stations arranged in three different sections: input/output, BAM-in-light and BAM-in-dark. The input/output section manages the input of the lead containers (8 tonnes a day) and the output of bricks (1000 a day). In the BAM-in-light section (a class 10,000 clean room) the lead containers are automatically opened and pallets of lead are dispatched towards the dark room. In the BAM-in-dark room (class 10,000 and red light) the bricks, each comprising 57 emulsion sheets interleaved with 56 lead plates, are piled up under a pressure of 3 bar. This operation is performed in parallel by five piling/pressing stations, each using two anthropomorphic robots and a custom-built press machine. The brick pile has to be aligned within 50 μm precision, while its components must be handled in such a way as to guarantee 10 μm flatness. The bricks are then wrapped with aluminium adhesive tape by a large anthropomorphic robot. Once the bricks are light-tight they are sent back to the BAM-in-light section where their dimensions are checked, they are equipped with Teflon skates (to reduce friction during insertion into the detector) and a CS box, and labelled.

The bricks are stored in a transport cage – the drum – which brings them in groups of 234 to OPERA, where the BMS positions them in the walls. This must be done simultaneously on both sides of the detector to maintain a balance, and so requires two independent BMS units. Each BMS comprises a 10 m high portico that can slide along the side of the two super-modules. A platform on the portico moves vertically to reach the different parts of the walls, which it accesses with a lift bridge with micrometre accuracy. The platform has a rotating buffer disk (or carousel) hosting 32 bricks and with devices for inserting the bricks (the pusher) and extracting them. The latter is a small vehicle with a vacuum (VV) sucker on the front.
To place bricks into a wall, the drum is placed on a loading station, and bricks are loaded into the BMS. They are then pushed one at a time on the lift bridge with the pusher, until a row of 26 bricks has been inserted and aligned to its nominal position in the semi-wall. This operation is repeated for each wall layer until the 64 layers are complete. Each BMS can handle about 500 bricks a day, following the BAM speed. It will take a year to fill the detector.
During extraction, the VV approaches the bricks on the appropriate wall layer through the lift bridge. The bricks are extracted by suction one by one onto the carousel until the selected one is found. The remaining bricks are then pushed back into the wall together with a replacement brick from the periphery of the target, which becomes slowly smaller with time.
The analysis chain
The bricks with neutrino interactions are placed in the drums and transported to a brick-handling area where the CS is removed and processed while the bricks wait for the analysis to confirm an event. If an interaction is not confirmed, the brick is equipped with a new CS box and placed back in OPERA. However, if the analysis confirms an event, the brick is brought to the external LNGS laboratory, exposed for a day to cosmic rays for alignment and then disassembled. The films are stamped with an identifier and reference marks, developed with an automatic system with several parallel processing chains as in a big photographic laboratory, and dispatched to the scanning laboratories. (The scanning of the CS is performed locally to provide fast feedback.)
A charged particle crossing an emulsion layer ionizes the medium along its path, leaving a sequence of aligned silver grains, with a linear size of about 0.6 μm. Typically, a minimum ionizing particle leaves about 30 grains in 100 μm. Observing with a high-magnification optical microscope, silver grains appear as black spots on a bright field. To reconstruct particles’ trajectories, the computer-driven microscope adjusts the focal plane of the objective lens through the whole thickness of the emulsion, obtaining an optical tomography at different depths of each field of view with typically one image every 3 μm. Each image is filtered and digitized in real time to recognize track grains as clusters of dark pixels. A tracking algorithm reconstructs 3D sequences of aligned grains and extract a set of relevant parameters for each sequence. The position accuracy obtained for the reconstructed tracks is about 0.3 μm, with a resulting angular resolution of about 2 mrad – adequate for reconstructing the tau-decay topology.
Matching tracks in the two CSs are extrapolated in the most downstream foil of the brick and followed plate by plate until the interaction vertex is located. A plate-by-plate alignment procedure is applied so that tracks can be followed back with a precision of few micrometres and the search for each track can be performed in one field of view (about 300 μm × 300 μm). Once the vertex has been located, a volume scan (about 5 mm × 5 mm in several consecutive plates) around the interaction point is done. All track segments that are found in this volume are recorded. Vertex reconstruction algorithms are applied to classify the event and search for particle decay topologies. Taking advantage of cosmic-ray tracks, recorded during the deliberate exposure of the bricks after their extraction, sub-micrometre precision can be reached on the reconstructed vertex, as required for detecting kink topologies with angles of a few milliradians and for the kinematical analysis of the event.
Commissioning and running
OPERA was proposed in 2000, with installation starting in May 2003; it detected the first neutrinos from CNGS on 18 August 2006. This first run was of 11 days, with 5 days of equivalent beam time. It was devoted to the final commissioning of the electronic detectors (target trackers and spectrometers) with data-taking in global mode, to check the synchronization of the OPERA and CNGS clocks and to test the reconstruction algorithms. The detector collected more than 300 neutrino interactions, with a live-time greater than 95%. These interactions mainly occurred in the rock and in the iron of the two magnets.
The August run was conceived to tune the electronic detector performance with CNGS neutrino interactions without bricks. OPERA has now started the next phase, which aims to observe neutrino interactions in the bricks. Brick production and insertion started in the second half of September, ramping up to 1000 a day. A first test run of three weeks with some thousand bricks exposed to CNGS is scheduled to begin in mid-October. Then the full tau search will proceed with the run in 2007.
Researchers foresee the experiment running for five years, with an integrated fluence of 2.25 × 1020 protons on the CNGS target. OPERA is sensitive to practically all of the tau-decay modes, with very low background levels: overall a total background of 1 event is expected. If νμ→ντ_oscillations occur, the average number of detected signal events will be 8.0–21.2 in the Super-Kamiokande 90% CL region and corresponds to 13.9 events for the best-fit value (Δm2 = 2.5 × 10-3 eV2, full mixing, Walter and Inoue 2006). OPERA can also search for_νμ→νe_oscillations with improved sensitivity on the still-unknown mixing angle θ13, compared with the best world limit obtained by the reactor experiment CHOOZ in 1998.