The latest international symposium on nuclear astrophysics showed that the field is rapidly evolving, with new observations from astronomy and nuclear physics.
Understanding our universe from basic physics is an ambitious goal involving many disciplines in physics. One key ingredient is nuclear astrophysics, with its focus on explaining energy production and chemical evolution in the universe – topics that are coupled through nuclear reactions that transform elements and may also release energy. The first overview of the synthesis of elements was about 50 years ago, with the work of Geoffrey and Margaret Burbidge, Willy Fowler and Fred Hoyle, and, independently, Al Cameron. Although there had been some important work earlier in the 20th century, this was the defining moment for nuclear astrophysics.
At the end of June, nearly 250 astronomers, astrophysicists, cosmologists and nuclear physicists met at CERN for the ninth Nuclei in the Cosmos meeting to summarize the status of the field. Organized by a team from the Isotope Separator On Line (ISOLDE) and Neutron Time-of-Flight (n_TOF) facilities at CERN, it was dedicated to the memory of Al Cameron, Ray Davis and John Bahcall, all of whom have recently died and had played major roles in helping to understand the production and role of nuclei in the cosmos.
Nuclei, of course, consist of smaller particles and the meeting reviewed recent developments in cosmology and their possible connection to particle physics. While at one time particle physics provided input for calculating the abundances of elements created during the early universe in Big Bang nucleosynthesis, nowadays the results from the Wilkinson Microwave Anisotropy Probe yield the baryonic density of the universe, which is used in calculating the abundances. However some problems in reconciling observations and calculations for the primordial elements remain, in particular for the two stable lithium isotopes, 6Li and 7Li.
Analysing nuclear ashes
Optical observations of stars reveal their element abundances. Old stars are metal-poor (following the convention in astronomy that all elements above helium are metals) and the heavy elements in them appear to be made exclusively by rapid neutron capture – the r-process. Only later in galactic evolution does the s-process – slow neutron capture – begin to contribute as well. The relative abundances for elements above barium fit well with r-process abundances deduced for solar-system material, but for lighter elements there are differences that could indicate the presence of a second (“weak”) r-process. The coming years should bring clarification as the amount of observational data will increase significantly owing to the large-scale surveys, the Hamburg/ESO R-process Enhanced Star survey and the Sloan Digital Sky Survey.
Another fruitful source of abundance data comes from presolar grains embedded in primitive meteorites. Here a recent breakthrough has been the extraction of isotopic ratios for many different grains. Such detailed information about isotope abundance helps in constraining the astrophysical conditions in which the grains were formed.
Refinements in knowledge of element abundances are not restricted to distant stars. An improved modelling of the solar atmosphere indicates that solar abundances of most “metals” should be decreased by more than a third. This change arises from a more careful and more dynamic treatment of the outer solar layers.
More direct evidence for nuclear processes in stars comes from the observation of the radioactive isotopes that are produced. High-resolution gamma-ray spectrometers have operated in space for some years, for example on the INTEGRAL satellite (figure 1). Several galactic radioactive decays have been observed through their gamma-emission lines, such as those of 26Al, 44Ti and 60Fe, although the decay of 22Na and positron emission both remain to be seen. Many of the radioactive isotopes found on Earth also stem from stellar events. A recent addition to the list is 60Fe, which has been identified in deep-ocean material by the highly sensitive accelerator mass spectrometer technique, indicating that a supernova exploded near the Earth about 2.4 million years ago.

Understanding stellar events
Modelling the evolution of stars and their sometimes violent end requires the coordinated work of many people. It is rather like assembling a giant multi-dimensional jigsaw puzzle, but one in which the pieces have first to be found. Some researchers concentrate on finding these pieces, while others focus on how to fit them together to form a coherent picture. However, we have still not identified the important ingredients for all stellar events.
For normal, “quiet” stellar burning, the nuclear reactions take place between stable isotopes, but with extremely small cross-sections that are hard to reproduce in the laboratory. Major efforts during the past decade have improved the situation, and participants at the meeting heard of progress on one of the remaining challenges, the reaction 12C(α,γ)16O, which is a key reaction for the processes responsible for the production of many elements.
At higher temperatures reaction rates increase and reactions involving radioactive nuclei need also to be known. A major highlight at the meeting in terms of nuclear data is a recent good estimate of the reaction rate for the key radiative alpha-capture process on 15O, which has been pursued for more than 20 years. The direct measurement of radiative proton-capture on 26gAl and alpha-capture on 40Ca was also presented. Lastly, reaction rates for several neutron-induced reactions were reported; these will have important implications for understanding the synthesis of elements heavier than iron – the “neutron capture elements”.
Very high temperatures belong to the domain of cosmic explosions – novae, supernovae, X-ray bursts and gamma-ray bursts – which have a fascinating history and rightly continue to attract attention. Numerical results presented at the meeting indicate that first-generation nova explosions occur at higher temperatures than classical novae. They could therefore be more important players in the early universe, but more studies are needed to confirm this.
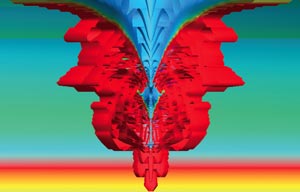
A highlight of the conference was the presentation of successful computer simulations of supernova explosions (figure 2). Here, the key new ingredient is to follow the two-dimensional hydrodynamic evolution to long durations after the core-collapse, when the inner part has become a highly non-spherical object with significant fluctuations. The violent conditions in a supernova are perfect for cooking elements and the meeting heard about a new mechanism, the np-process, in which the strong neutrino fluxes play a more versatile role in the nucleosynthesis than imagined earlier. An exciting report on quantitative calculations of the r-process suggested that nuclear fission, and in particular neutron-induced fission, might play a very important role for the dynamics in later stages of the r-process.
It was clear from the meeting that nuclear astrophysics is rapidly evolving. The next meeting in the series will provide another snapshot of the status of the field, when it takes place in the US in 2008, hosted by the Michigan State University/National Superconducting Cyclotron Laboratory and the Joint Institute for Nuclear Astrophysics.