A new experiment gets going using Fermilab’s upgraded neutrino beam.
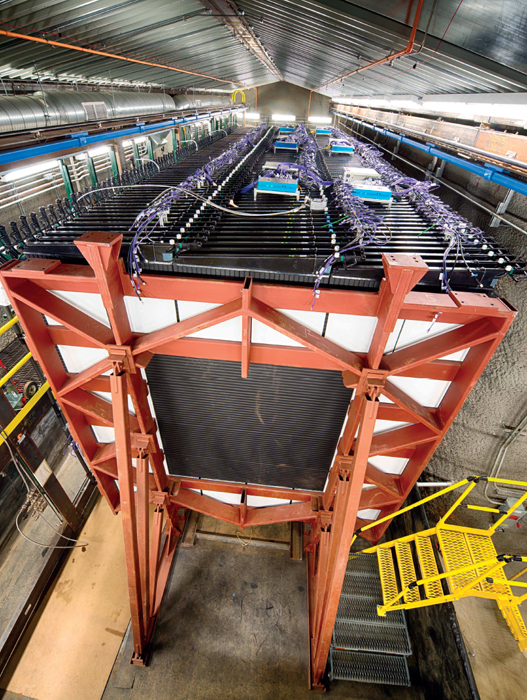
Image credit: Fermilab Visual Media Services.
NOvA, Fermilab’s new flagship neutrino-oscillation experiment, has recorded its first neutrinos and is now poised to make precision measurements of electron-neutrino (νe) appearance and muon-neutrino (νμ) disappearance. These data will help to unravel remaining unknowns in understanding neutrino masses and mixing. In the now standard picture of neutrinos, the three electroweak flavour states (νe, νμ and ντ) are mixtures of the mass eigenstates (ν1, ν2 and ν3) related by a unitary matrix that is parameterized by three mixing angles and a charge-parity (CP) violating phase. Neutrinos are produced and detected in flavour eigenstates but propagate in mass eigenstates. Interference among the mass states means that a neutrino created in a definite flavour state can later be detected in a different flavour state. This oscillation probability is determined by the sizes of the mixing angles, the splittings in the neutrino masses, the energy of the neutrino and the distance it has travelled. Measurements of the oscillation probabilities of neutrinos of known energy that travel a known distance reveal the underlying mass-splittings and mixings.
Thanks to experiments using neutrinos produced in the Sun, in the atmosphere, at particle accelerators and in nuclear reactors, researchers have found out a great deal about neutrino masses and mixing. We know that two neutrinos are relatively close in mass and that the third is relatively far away in mass. We know that the mixing angles are all relatively large, in contrast to mixing angles in the quark sector, which are small. We also know that the two neutrinos that are relatively close in mass contain most of the electron-neutrino flavour, and that the third is a nearly equal combination of muon and tau flavour. However, we do not know if the third mass eigenstate is composed of more νμ or ντ, or if a new symmetry keeps these two contributions equal. We do not know if neutrinos violate CP symmetry, and we do not know the ordering of the neutrino masses.
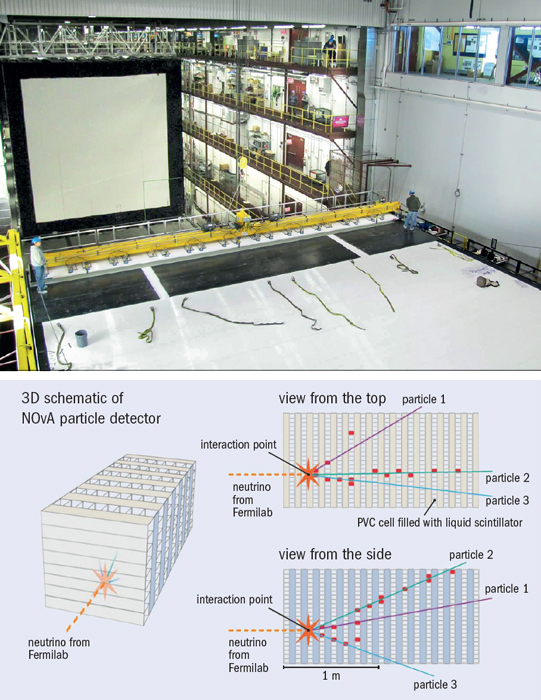
Image credits: Fermilab Visual Media Services.
Neutrinos could follow a normal hierarchy, with most of the νe content contained in the lightest two states, or they could follow an inverted hierarchy with the νe content predominantly in the heaviest two states. The neutrino-mass hierarchy is one specific prediction of different grand-unification theories, with implications for cosmological measurements of the absolute scale of neutrino mass. The hierarchy, in combination with results from neutrinoless double-beta decay experiments, plays an important role in determining the Dirac or Majorana nature of the neutrino.
NOvA will use two detectors to measure oscillation probabilities in Fermilab’s NuMI (Neutrinos at the Main Injector) muon-neutrino beam. When neutrinos travel the 810 km between Fermilab and Ash River, Minnesota, through the crust of the Earth, scattering of νe on atomic electrons can either enhance or suppress the oscillation probability, depending on the mass hierarchy. The effect is opposite in neutrinos compared with antineutrinos, so by comparing the oscillation probability measured in neutrinos with that measured with antineutrinos, NOvA can determine the mass hierarchy, resolve the nature of ν3, and begin the study of CP violation in neutrinos.
To achieve these goals, NOvA requires an intense neutrino and antineutrino source. NuMI had routinely delivered 320 kW of beam power to the MINOS and MINERvA experiments during operation of the Tevatron. However, with Tevatron operations now ended, the accelerator complex has been reconfigured to provide twice the beam power to the NuMI beamline. During a shutdown of a year and a half starting in the spring of 2012, a major RF upgrade in the Main Injector was accomplished, reducing its cycle time from 2.2 s to 1.67 s. Additionally, the Recycler ring, which was key to antiproton generation for the Tevatron, was converted to a proton accumulator so that protons can be integrated and stored during the Main Injector ramp from 8 GeV at injection to 120 GeV.

At the same time, the NuMI beamline underwent a transformation to accommodate the higher proton intensities required for NOvA. The neutrino target and focusing horns were replaced and repositioned. The new beam provides higher-energy neutrinos on-axis, but at 14 mrad off the beam axis – where the NOvA detectors are located – the neutrino energy spectrum is peaked narrowly at 2 GeV, the perfect energy for the long-baseline oscillations that NOvA will study.
Beam began circulating again in the Main Injector in September 2013 and work started on commissioning the new accelerator in the Recycler ring. The Recycler is now normally included in operations, and work is underway to “slip stack” routinely in this new machine – a delicate manoeuvre where one bunch is injected then shifted to a different orbit to make room for a second bunch in the same RF bucket. Once the two bunches are merged, they are accelerated together. This work is expected to bring the NuMI intensity to 450 kW by the end of the year, and ongoing upgrades to the Booster ring that feeds this complex are expected to bring the intensity to 700 kW within another year. Since coming back up from the shutdown, the complex has achieved a peak beam power of more than 300 kW and delivered almost 2.5 × 1020 protons to NOvA and the other two neutrino experiments sharing the beam, MINOS+ and MINERvA.
The NOvA detector must be big to overcome the small size of the neutrino-interaction cross-section and the 810 km distance from the neutrino source
In addition to an intense beam, NOvA also requires a massive far detector and a functionally identical near detector. Like all neutrino detectors, the NOvA detector must be big to overcome the small size of the neutrino-interaction cross-section and the 810 km distance from the neutrino source. Being big, however, is not enough. The detector must also be highly segmented to prevent the numerous cosmic rays that cross the detector from interfering with neutrino events from Fermilab. Furthermore, to separate electromagnetic showers from electron-neutrino events from similar showers from other sources, especially the decays of π0 mesons, heavy materials of high atomic number (Z) such as steel – which are normally used to build large structures – have not been employed.
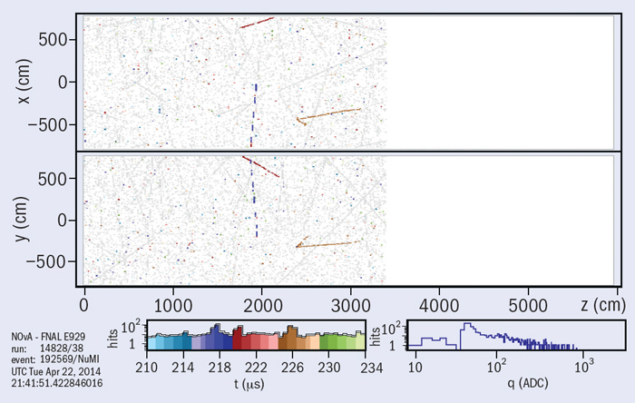
The NOvA detectors (figures 1 and 2) are a unique solution to the particular challenges of observing νe appearance using the NuMI neutrino beamline. The NOvA far detector is a 14,000 tonne detector, using 9000 tonnes of liquid scintillator – the largest quantity of liquid scintillator ever produced for a physics experiment – to record the tracks of charged particles. The scintillator is contained in a 15.6 × 15.6 × 60 m3, 5000-tonne PVC structure constructed from modules assembled at a factory operated by collaborators at the University of Minnesota. A crew of more than 700 undergraduate students directed by 10 full-time staff members ran the factory. These pieces were shipped to the Ash River Laboratory in Northern Minnesota, where another 45 full-time staff members built the 28 free-standing blocks that make up the detector. The 190-tonne blocks were constructed horizontally on an enormous table, which later pivoted them into a vertical position and placed them in the experimental hall.
In addition to containing the scintillator, the PVC structure segments the detector into 4 cm × 6 cm × 15.6 m channels. Light produced in these channels by the charged particles that traverse them bounces 10 times, on average, before it is captured in a wavelength-shifting fibre. To ensure that enough light is captured in the fibre, a special PVC formulation with enhanced reflectivity had to be developed. The large size of the detector and the large number of channels required more than 10,000 km of wavelength-shifting fibre – enough to stretch from the supplier in Japan to the Ash River Laboratory in a single unbroken thread.
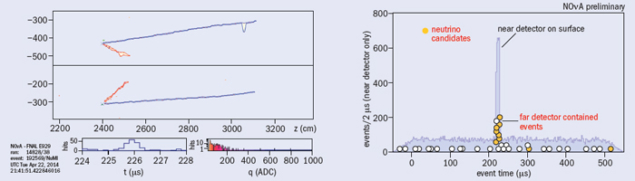
This large-scale assembly project is now finished. The last detector block was put in place in February of this year and the last of the 11 million litres of scintillator made for the experiment was delivered in April. While the task of outfitting the detector with electronics is continuing through the summer, the experiment recorded its first neutrino event in November last year, and has analysed millions of cosmic-ray tracks. This analysis has verified that the scintillator, PVC, fibre and electronics work together as designed to move the scintillation light from the far end of the detection channels to where it can be recorded. As figure 3 shows, the efficiency for detecting a minimum-ionizing particle crossing a cell at the furthest end from the read-out is above 90%, which is key to the tracking and particle-identification performance of the detector.
First events
Cosmic-ray interactions are an excellent source for detector calibration, but they are also a potential background to the neutrino selection. While the NuMI beam is delivered in regular bursts, 10 μs in duration, the high cosmic rate on the surface means that about 1.5 cosmic interactions are expected in the detector during the spill. On the other hand, after oscillations, a NuMI neutrino interacts in the far detector once every 12,000 spills, or only about once every four hours. Containment and directional cuts suppress the cosmic rate by about a factor of 105, with only minimal loss of neutrino events. Figure 4 shows a charged-current νμ interaction identified in the NOvA far detector, along with two cosmic-ray muons zipping through during the beam spill. Figure 5 shows the same event, reconstructed, as well as a timing distribution of other neutrino candidates found in the far detector. The neutrino candidates pile up at the arrival time measured in the NOvA prototype detector delayed by the neutrino flight time between the two sites, confirming that NOvA can identify neutrinos among the cosmic-ray backgrounds.
In May, one sixth of the full near detector was turned on for the first time, and neutrinos were seen in the first spills
While relatively simple cuts can be used to separate beam neutrino events from cosmogenic events, further suppression of cosmic rays is required to achieve the oscillation physics goals. Multivariate event-selection algorithms tuned to recognize the differing topologies of νμ and νe charged-current and and neutral-current interactions suppress the cosmic-ray background rate by a further two orders of magnitude. Data collected when the beam is known to be off confirm that the necessary level of rejection can be achieved: the cosmic-ray background in a one-year exposure is predicted to be one event in the νμ sample and 0.5 events in the νe sample, well below the expected signal rates of 75 and 15 neutrinos in these samples.
The NOvA collaboration is now eagerly awaiting data from the near detector, which are needed to measure the beam composition and energy spectrum before oscillations have developed. The near-detector data will set the background expectation in the far detector for the νe appearance channel, and determine the unoscillated event rate as a function of energy for the νμ disappearance channel. In May, one sixth of the full near detector was turned on for the first time, and neutrinos were seen in the first spills. NOvA researchers are looking forward to an exciting summer.