A workshop in Marseilles focused on the search for exotic physics in the era of large-scale neutrino telescopes and the LHC.

Image credit: NSF/S Lidström
It is more than six years since Uppsala University was host to the first Workshop on Exotic Physics with Neutrino Telescopes. Since then, the large neutrino telescopes IceCube and ANTARES have been completed and indirect searches for dark matter, monopoles and other aspects of physics beyond the Standard Model are proceeding at full strength. Indeed, some theoretical models have already been called into question by recent results from these detectors. Meanwhile, searches for dark-matter candidates and indications of physics beyond the Standard Model in experiments at the LHC have set stringent constraints on many models, complementing those derived from the neutrino telescopes. The time was therefore ripe for a second workshop, with the Centre for Particle Physics of Marseilles (CPPM) as host, bringing together 47 experts on 3–6 April.
Dark matter
Review talks on supersymmetric dark-matter candidates and the status of experimental searches opened the first day’s sessions. Supersymmetry – still a well motivated candidate for physics beyond the Standard Model – has been put to the first serious tests at the LHC. The discovery there of a Higgs boson at a mass of 126 GeV can be seen either as just another confirmation of the Standard Model or as a first glimpse of physics beyond it. The lack of evidence so far for supersymmetry from direct searches at the LHC raises the limits of supersymmetric particle masses to the scale of tera-electron-volts and has implications for the dark-matter candidates arising in supersymmetric models. The current preferred mass-range for the lightest, stable neutralino is in the region of hundreds of giga-electron-volts. This is good news for neutrino telescopes, which – by design – are sensitive to high-energy particles. The downside is that the predicted rates from annihilation of neutralinos accumulated in heavy celestial objects are low if the constraints from the Wilkinson Microwave Anisotropy Probe and the LHC are taken into account. Only a handful of minimal supersymmetric Standard Model variants predict rates in cubic-kilometre neutrino telescopes that are of the order of 100 events per year or higher.
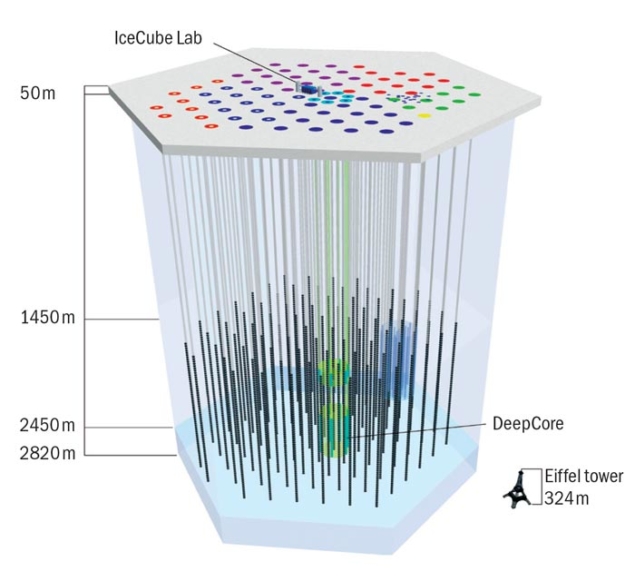
Image credit: IceCube collaboration
However, the neutralino in minimal supersymmetry is not the only viable candidate for dark matter. In models with R-parity violation, a long-lived but unstable gravitino with a mass between a few and a few hundred giga-electron-volts could be a component of the dark matter in the halo of galaxies. Neutrinos of any flavour can be produced in gravitino decay and can be detected by neutrino telescopes. A feature of gravitino dark matter is that it would leave no signal in direct-detection experiments because the cross-section for the interaction between a gravitino and normal matter is suppressed by the Planck mass to the fourth power.
Models with extra dimensions of sizes in the range 10–3–10–15 m can also provide dark-matter candidates. Extra dimensions can be accommodated (or even required) in supersymmetry, string-theory or M-theory, where they give rise to branons – weakly interacting and massive fluctuations of the field that represents the 3D brane on which the standard world lives. As stable and weakly interacting objects, branons make a good candidate for dark matter, following the usual scenario: relic branons left over after a freeze-out period during the evolution of the universe accumulate gravitationally in the halos of galaxies, where they annihilate into Standard Model particles that can be detected by gamma-ray telescopes, surface arrays or neutrino telescopes.

Image credit: Anna Obertacke, Wuppertal
From the experimental side, the IceCube, ANTARES, Baikal, Baksan and Super-Kamiokande collaborations presented their latest results on the search for dark matter from different potential sources – the Sun, the Galaxy or nearby galaxies. Their search techniques are similar and based on looking for an excess of neutrinos over the known atmospheric-neutrino background from the direction of the sources. DeepCore, the denser array in the centre of IceCube, which was not part of the original design, has proved extremely useful in lowering the energy reach of the detector. It has opened up the possibility of pursuing physics topics that would otherwise be impossible with a detector designed for tera-electron-volt neutrino astrophysics. Using the surrounding strings of IceCube as a veto, starting and contained tracks can be defined, therefore turning IceCube into a 4π detector with an energy threshold of around 10 GeV, with access to the Galactic centre and the whole Southern Sky.
However, none of the experiments report any excess, and upper limits on the neutrino flux and on the cross-section for interactions between weakly interacting massive particles (WIMPs) and nucleons have been calculated over an ample range of WIMP masses, from about 1 GeV (Super-Kamiokande) to 10 TeV (IceCube). An example of the long-term search capability, as well as consistency in data analysis, was presented for the Baksan experiment. Although it has the smallest of the detectors mentioned above, it has gathered data over 24 years, from 1978 to 2009.
Monopoles, nuclearites and more
Monopoles and heavy, highly ionizing particles leave a unique signal in a neutrino telescope: a strong light-yield along the path of the particle, which is much more intense than the usual track-pattern of a minimum-ionizing muon. If the particle is nonrelativistic, then the separation of such a signal from relativistic muons traversing the detector is even easier. However, dedicated online or offline triggers are needed because for a nonrelativistic particle, light is deposited in the detector over a time of up to tens of milliseconds, instead of a few microseconds for a relativistic muon.
The best limit for fast (β > 0.75) monopoles, at a level of about 3 × 10–18 cm–2 s–1, was presented by the IceCube collaboration using data from its 40-string configuration, although the ANTARES limit – at a level of around 7 × 10–17 cm–2 s–1 – remains the best so far, at between 0.65 < β < 0.75. However, the sensitivity of the full IceCube detector could extend to β = 0.60 and reach a level of between 2 × 10–18 cm–2 s–1and 10–17 cm–2 s–1 in a one-year exposure, depending on the assumptions on the monopole spin. Results are expected soon, when the ongoing data analysis is finalized.
The Super-Kamiokande collaboration presented a novel way to search for monopoles using the Sun as the target. The idea is that super-heavy monopoles that have been gravitationally trapped in the Sun will induce proton decay along their orbits. Neutrinos with an energy of tens of mega-electron-volts will then be emitted by the decays of the muons and pions produced as the protons decay. This is a low-energy signal that is well below the threshold of large-scale neutrino telescopes but for which Super-Kamiokande has sensitivity. Indeed, this experiment provides the best limit so far on the flux of super-heavy monopoles in the range 10–5 < β < 10–2. At the other end of the kinematic spectrum, radio-Cherenkov detectors such as RICE and ANITA provide the best limits for ultrarelativistic monopoles of intermediate mass, at the level of 10–19 cm–2 s–1.
Another bright signature, although from a different process, is produced by slowly moving heavy nuclearites. These massive stable lumps of up, down and strange quarks could be detected in neutrino telescopes through the blackbody radiation emitted by the overheated matter along their path. From the analysis of 310 days of live time in the years 2007–2008, the ANTARES collaboration reported a flux limit at the level of 10–17 cm–2 s–1 sr–1 for nuclearite masses larger than 1014 GeV and β around 10–3. Indeed, the limit improves previous results from the MACRO experiment by a factor of between three and an order of magnitude, depending on the nuclearite mass.
The atmosphere, acting as a target for ultra-high-energy cosmic rays, can be a useful source for searches of physics beyond the Standard Model
The atmosphere, acting as a target for ultra-high-energy cosmic rays, can be a useful source for searches of physics beyond the Standard Model. The interaction of a cosmic ray of energy around 1011 GeV with a nucleon in the atmosphere takes place at a much higher centre-of-mass energy than is achievable in accelerator laboratories and a wealth of physics can be extracted from such collisions. Supersymmetric particles can be produced in pairs and, except for the lightest, they can be charged. Even if unstable, they can, because of the boost in the interaction, reach the depths of a detector and emit Cherenkov light as they traverse an array. The signature is two minimum-ionizing, parallel, coincident tracks separated by more than 100 m. These types of searches are being carried out by the two large neutrino-telescope collaborations, IceCube and ANTARES.
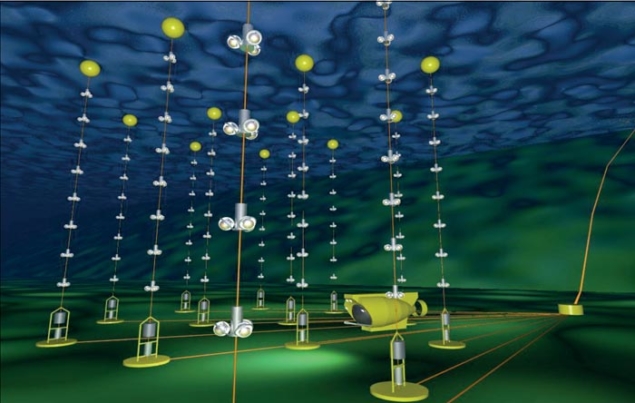
Image credit: F Montanet, CNRS/IN2P3 and UJF for Antares/produced with POV-ray
The same interactions of cosmic rays with the atmosphere can also be used to probe non-standard neutrino interactions arising from the effects of tera-electron-volt gravity and/or extra dimensions. At high energies, neutrino interactions with matter may become stronger and the atmosphere can become opaque to neutrinos with energies of peta-electron-volts. A signature in a neutrino telescope would be an absence of regular neutrinos with ultra-high energies accompanied by an excess of muon bundles at horizontal zenith angles. The same effect would take place with a cosmogenic neutrino flux – that is, the flux of neutrinos produced by the interactions of ultra-high-energy cosmic rays with the cosmic microwave background radiation. In the absence of a discovery so far, this flux can be assumed to be at a level compatible with gamma-ray constraints from the Fermi Gamma-ray Space Telescope. The neutrino-nucleon cross-section will depend on the number of extra dimensions, ND, and a lack of events over the expected flux can be transformed into a limit on ND. However, the effect in neutrino telescopes with volumes of a cubic kilometre or so is not big. For values of ND not excluded by the LHC, fewer than one event a year is estimated for IceCube. Only with the larger radio arrays is the expected number of events of the order of 10 per year.
The recent two peta-electron-volt events announced by the IceCube collaboration have already been used to set stringent limits on the violation of Lorentz invariance. If strict Lorentz invariance does not hold, then neutrino bremsstrahlung of electron–positron pairs (ν → νe+e–) is possible, so extragalactic neutrinos would rapidly lose energy via such a process. This would lead to a depletion of the ultra-high-energy neutrino flux at the Earth. Assuming that the IceCube events are, indeed, extragalactic (that is, they have travelled of the order of megaparsecs from the sources to the Earth) and that the extragalactic high-energy neutrino flux is at most at the level of the current IceCube limit of 2 × 10–8 cm–2 s–1 sr–1, a limit can be set on Lorentz invariance violation, parameterized by the factor δ, defined as (dE/dp)2-1. Under these assumptions, the bound obtained from the two IceCube events is δ <10–18, which is orders of magnitude smaller than the current best limit of 10–13.
Even if conventionally produced, the absolute normalization of the atmospheric lepton spectrum is not well understood
High-energy atmospheric muons and neutrinos present a background to many of the topics discussed in the workshop. Even if conventionally produced, the absolute normalization of the atmospheric lepton spectrum is not well understood – in particular the contribution from prompt charm decays. Calculations of an atmospheric lepton component, which is rarely considered, from the decays of unflavoured mesons (η, η’, ρ, ω, φ), were presented at the workshop. These mesons decay rapidly to μ+μ– pairs and in very-high-energy cosmic-ray interactions the products of the decays can be the dominant muon flux at energies above 106 GeV, forming a background that must be taken into account in exotic searches.
One of the unexpected developments in the field since the first ideas of building neutrino telescopes has been their use in neutrino-oscillation physics. On one hand, the detectors can probe oscillation physics at energies not reachable by the smaller detectors. On the other, an aggressive plan to lower the energy threshold of IceCube and the proposed KM3NeT array to the few-giga-electron-volt region is underway, and IceCube has already produced physics results with its low-energy subarray, DeepCore. Plans to build megatonne water-Cherenkov detectors with a giga-electron-volt energy threshold – PINGU at the South Pole and ORCA in the Mediterranean – were also discussed in the workshop. These detectors consist of about 20–50 strings of optical modules with an inter-string separation of 20 m, to be compared, for example, with the 125 m inter-string separation of IceCube or the 70 m inter-string separation of DeepCore. Such detectors may address the issue of the neutrino mass hierarchy at a relatively low cost and on a short timescale because the technology exists already and the deployment techniques are the same as in IceCube and ANTARES.