Options for a Higgs factory include a bigger version of LEP.
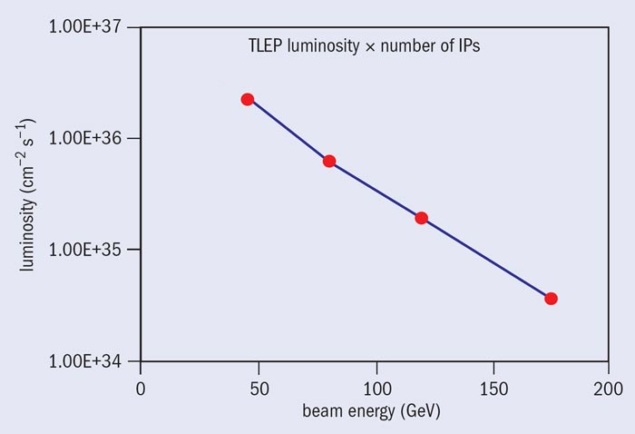
The discovery of a Higgs boson by the ATLAS and CMS collaborations at the LHC has opened new perspectives on accelerator-based particle physics. While much else might well be discovered at the LHC as its energy and luminosity are increased, one item on the agenda of future accelerators is surely a Higgs factory capable of studying this new particle in as much detail as possible. Various options for such a facility are under active consideration and circular electron–positron (e+e–) colliders are now among them.
In a real sense, a Higgs factory already exists in the form of the LHC, which has already produced millions of Higgs bosons and could produce hundreds of millions more with the high-luminosity upgrade planned for the 2020s. However, the experimental conditions at the LHC restrict the range of Higgs decay modes that can be observed directly and measured accurately. For example, decays of the Higgs boson into charm quarks are unlikely to be measurable at the LHC. On the one hand, decays into gluons can be measured only indirectly via the rate of Higgs production by gluon–gluon collisions and it will be difficult to quantify accurately invisible Higgs decays at the LHC. On the other hand, the large statistics at the LHC will enable accurate measurements of distinctive subdominant Higgs decays such as those into photon pairs or ZZ*. The rare decay of the Higgs into muon pairs will also be accessible. The task for a Higgs factory will be to make measurements that complement or are even more precise than those possible with the LHC.
Attractive options
Cleaner experimental conditions are offered by e+e– collisions. Prominent among other contenders for a future Higgs factory are the design studies for a linear e+e– collider: the International Linear Collider (ILC) and the Compact Linear Collider (CLIC). In addition to running at the centre-of-mass energy of 240 GeV that is desirable for Higgs production, these also offer prospects for higher-energy collisions, e.g. at the top–antitop threshold of 350 GeV and at 500 GeV or 1000 GeV in the case of the ILC, or even higher energies at CLIC. These would become particularly attractive options if future, higher-energy LHC running reveals additional new physics within their energy reach. High-energy e+e– collisions would also offer prospects for determining the triple-Higgs coupling, something that could be measured at the LHC only if it is operated at the highest possible luminosity.

There has recently been a resurgence of interest in the capabilities of circular e+e– colliders being used as Higgs factories following a suggestion by Alain Blondel and Frank Zimmermann in December 2011 (Blondel and Zimmermann 2011). It used to be thought that the Large Electron–Positron (LEP) collider would be the largest and highest-energy circular e+e– collider and that linear colliders would be more cost-efficient at higher energies. However, advances in accelerator technology since LEP was designed have challenged this view. In particular, the development of top-up injection at B factories and synchrotron radiation sources, as well as advances in superconducting RF and in beam-focusing techniques at interaction points, raise the possibility of achieving collision rates at each interaction point at a circular Higgs factory that could be more than two orders of magnitude larger than those achieved at LEP. Moreover, it would be possible to operate such a collider with as many as four interaction points simultaneously, as at LEP.
The concept for a circular e+e– collider that has been most studied is TLEP, which would be installed in a tunnel some 80–100 km in circumference. This would be capable of collisions at 350 GeV in the centre of mass, while the specifications call for a luminosity of 1034 cm–2 s–1 at this energy at each of the four interaction points. With conservative technical assumptions, the corresponding luminosity at a centre-of-mass energy of 240 GeV would exceed 4 × 1034 cm–2 s–1 at each interaction point, as figure 1 shows (Koratzinos et al. 2013). It is encouraging that previous circular e+e– colliders – such as LEP and the B factories – have established a track record of exceeding their design luminosities and that there are no obvious show-stoppers to achieving these targets at TLEP.
The design luminosity of TLEP would enable millions of Higgs bosons to be produced under clean experimental conditions
The design luminosity of TLEP would enable millions of Higgs bosons to be produced under clean experimental conditions. The Higgs mass could then be measured with a statistical precision below 10 MeV and the total decay width with an accuracy of better than 1.5%. Many decay modes, such as those into gluon pairs, WW*, ZZ*, and invisible decays could be measured with an accuracy of better than 0.2% and γγ decays to better than 1.5%. This would challenge the predictions of reclusive supersymmetric models, which predict only small deviations of Higgs properties from those expected in the Standard Model, as figure 2 shows.
One essential limitation on the ambition for such a collider is the overall power consumption. The largest, single energy requirement is for the RF acceleration system. Fortunately, because it would operate in continuous rather than pulsed mode, experience with LEP suggests that an overall efficiency above 50% should be attainable. The collision performances quoted here would require an RF power consumption of around 200 MW, to which should be added some 100 MW for cooling, ventilation, other services and the experiments. This is similar to the requirements of other major future accelerators at the energy frontier, such as the ILC and CLIC.
One attractive feature of circular e+e– colliders is that they could offer significantly higher luminosities at lower energies. For example, a total luminosity of 2 × 1036 cm–2 s–1 should be possible with TLEP running at the Z peak, and 5 × 1035 cm–2 s–1 at the W+W– threshold, which would offer prospects of data samples with the order of 1012 Zs and 108 W events. The statistical precision and the sensitivity to rare decays provided by such samples extend far beyond those envisaged in previous studies of Z and W physics, corresponding, e.g. perhaps to δsin2θW ˜ 10–6 and δmW ˜ 1 MeV. It will require both a major experimental effort to understand how to control systematic errors and a major theoretical effort to optimize the interpretation of the information obtainable from such data sets.
Although the baseline for TLEP is a tunnel with a circumference of 80–100 km, it is interesting to consider how the performance of a circular e+e– collider would scale with its circumference. Generally speaking, a smaller ring would be expected to have a lower maximum centre-of-mass energy, as well as lower luminosities at the energies within its reach. The lower limit of the range of ring sizes under consideration is represented by the LHC tunnel, with its circumference of 27 km. An e+e– collider in an LHC-sized tunnel could reach 240 GeV in the centre of mass – for Higgs studies with a luminosity above 1034 cm–2 s–1 at each interaction point – and could produce impressive quantities of Z bosons and WW pairs. It is difficult to imagine installing an e+e– collider in the LHC tunnel before the LHC experimental programme runs its full course but installation in a new tunnel would not be subject to such a restriction and interest in such a project has been expressed in various regions of the world.
One attractive option would be to envisage a future circular e+e– collider as part of a future, very large collider complex. For example, a tunnel with a circumference of 80–100 km could also accommodate a proton–proton collider capable of collisions at 80–100 TeV in the centre of mass, which would also open up the option of very-high-energy electron–proton collisions. This could be an appealing vision for accelerator particle physics at the energy frontier for much of the 21st century. Such a complex would fit naturally into the updated European Strategy for Particle Physics, which has recently been approved.