How a simple but audacious experiment opened up a new technique.
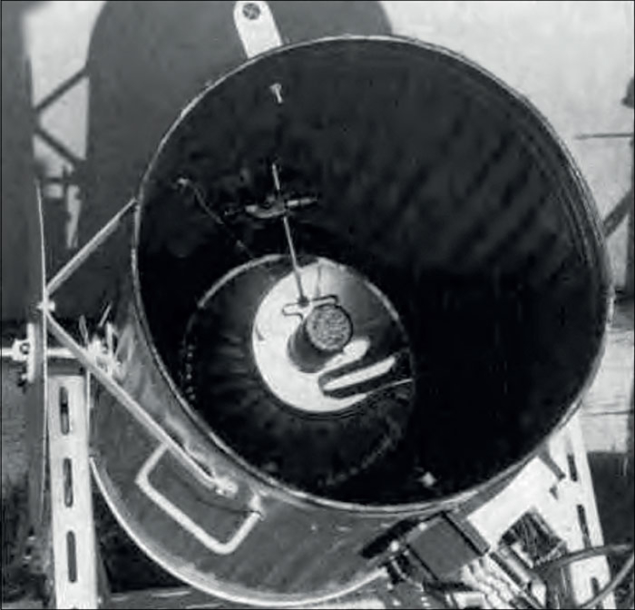
Sixty years ago, in September 1952, two young researchers at the UK’s Atomic Energy Research Establishment went out on a moonless night into a field next to the Harwell facility equipped with little more than a standard-issue dustbin containing a Second World War parabolic signalling mirror only 25 cm in diameter, with a 5 cm diameter photomultiplier tube (PMT) at its focus, along with an amplifier and an oscilloscope. They pointed the mirror at the night sky, adjusted the thresholds on the apparatus and for the first time detected Cherenkov radiation produced in the Earth’s atmosphere by cosmic rays (Galbraith and Jelley 1953).
William (Bill) Galbraith and John Jelley were members of Harwell’s cosmic-ray group, which operated an array of 16 large-area Geiger-Müller counters for studying extensive air showers (EAS) – the huge cascades of particles produced when a primary cosmic particle interacts in the upper atmosphere. Over several nights, by forming suitable coincidences between the Geiger-Müller array and their PMT, Jelley and Galbraith demonstrated – unambiguously – a correlation between signals from the array and light pulses of short duration (<200 ns) with amplitudes exceeding 2–3 times that of the night-sky noise. By cross-calibrating with alpha particles from a 239Pu source, they were further able to estimate that they were detecting three photons per square centimetre per light flash in the wavelength range of 300–550 nm. A new age of Cherenkov astronomy was born.
The sky at night
Five years before this observation, at a meeting of the Royal Society’s Gassiot Committee in July 1947 on “The emission spectra of the night sky and aurorae”, Patrick Blackett had presented a paper in which he suggested, for the first time, that Cherenkov radiation emitted by high-energy cosmic rays should contribute to the light in the night sky. Blackett estimated the contribution of cosmic-ray-induced Cherenkov light to be 0.01% of the total intensity, concluding: “Presumably such a small intensity of light could not be detected by normal methods.” Blackett’s work went largely unnoticed until a chance meeting at Harwell in 1952, which Jelley later recounted (Jelley 1986): “… hearing of our work on Cherenkov light in water, [Blackett] quite casually mentioned that … he had shown that there should be a contribution to the light of the night sky, amounting to about 10–4 of the total, due to Cherenkov radiation produced in the upper atmosphere from the general flux of cosmic rays.” Jelley continued: “Blackett was only with us a few hours, and neither he nor any of us ever mentioned the possibility of pulses of Cherenkov light, from EAS. It was a few days later that it occurred to Galbraith and myself that such pulses might exist and be detectable.”
The work of 1952 demonstrated the presence of short-duration pulses of light in coincidence with EAS but it did not prove that the light was, indeed, Cherenkov radiation. In particular, Galbraith and Jelley were aware that the light that they had observed could be also be produced either by bremsstrahlung or by recombination following ionization in the atmosphere. Thus, in the summer of 1953, they set out to establish the Cherenkov nature of the light pulses that they had observed.
Daunted by the vagaries of the British weather, they headed to the Pic du Midi observatory in France where, over six moonless weeks in July to September 1953, they carried out a series of experiments to determine the polarization and directionality of the light and also performed a rudimentary wavelength determination. This time they were equipped with four mirrors and two types of PMT. Conscious that the light-pulse counting rate would change with the noise level of the night sky, which in turn would depend on which part of the sky they were looking at, they devised a method of keeping the mean PMT current and, hence the noise, constant by using a small lamp next to the mirror.
Experimental conditions at the top of the mountain were challenging. EAS correlations were provided by requiring coincidences of signals from the PMTs with those from a linear array of five trays of Geiger-Müller counters, each tray 800 cm2 in area and aligned over almost 75 m – the positioning of these units was somewhat limited by the available space on the mountain (Galbraith and Jelley 1955). PMT pulses were recorded on an oscilloscope and subsequently photographed. Evidence for polarization of the observed light, a known characteristic of Cherenkov radiation, was clearly established by taking readings of a PMT with a polarizer placed over the PMT’s photocathode and calculating the ratio of the number of events seen when the polarizer was aligned parallel or perpendicular to the Geiger-Müller array. The result was a ratio of 3.0±0.5 to 1 for events seen in coincidence with two Geiger-Müller counter trays (Jelley and Galbraith 1955).
The two researchers also investigated the directionality of the observed light by plotting the coincidence rate of pulses seen in two light receivers (normalized accordingly) as a function of the angle between the two receivers. This experiment was done using pairs of receivers 1 m apart and was repeated with mirrors having different fields of view. The results fell between the two theoretical curves for Cherenkov and ionization light but they gave additional support for the premise that the light being observed was, indeed, Cherenkov light. In addition, the use of wide-band filters enabled Galbraith and Jelley to demonstrate that the light contained more blue light than green, which was another expected feature of Cherenkov radiation.
During their studies on the Pic du Midi, Jelley and Galbraith went on to explore the relationship between the light yield in the atmosphere and the energy of the shower, confirming, as expected, that larger light pulses were correlated with showers with higher particle densities. Finally, aware that their light receivers had both a considerable effective area and good angular resolution, they went on to search for possible point sources of cosmic rays in the night sky. The search yielded no statistically significant variations, and Galbraith and Jelley subsequently estimated that the receiver was sensitive to showers of energies of 1014 eV and above.
Following these studies in the early 1950s, it soon became apparent that use of the atmosphere as a Cherenkov radiator was a viable experimental technique. By the end of the decade, Cherenkov radiation in the atmosphere had been developed further as a means for studying cosmic rays – far away from the generally unsuitable British climate. In the Soviet Union, Aleksandr Chudakov and N M Nesterova of the Lebedev Physical Institute deployed a series of large-area Geiger counters along with eight light receivers at 3800 m in the Pamir Mountains to detect the lateral distribution of the Cherenkov light and thereby study the vertical structure of cosmic-ray showers. In Australia, around the same time, Max Brennan and colleagues of the University of Sydney used two or more mis-aligned light receivers to demonstrate the effects of Coulomb scattering of the charged particles in the cosmic-ray shower.
Meanwhile, at the International Cosmic Ray Conference in Moscow in 1959, Giuseppe Cocconi made a key theoretical prediction – that the Crab Nebula should be a strong emitter of gamma rays at tera-electron-volt energies. This stimulated further work, both by a British–Irish collaboration that included Jelley, and by Chudakov and his colleagues. The work at the Lebedev Physical Institute led in the early 1960s to the construction of the first air-Cherenkov telescope, with 12 searchlight mirrors, each 1.5 m in diameter and mounted on railway cars at a site in the Crimea close to the Black Sea.
The legacy
So, just a decade after the initial pioneering steps by Galbraith and Jelley, the first operational air-Cherenkov telescope had been built, setting in motion a chain of events that would ultimately lead in 1989 to the observation of gamma rays from the Crab Nebula by Trevor Weekes and colleagues at the Whipple telescope in the US. This breakthrough came nearly 25 years after Weekes had worked with Jelley in a collaboration between AERE and the University College Dublin, making the first attempts to detect gamma rays from quasars – a feat achieved only recently by the MAGIC air-Cherenkov telescope in the Canary Islands. Now, researchers around the world are teaming up to build the most sensitive telescope of this kind yet – the Cherenkov Telescope Array (Cherenkov Telescope Array is set to open new windows).
In writing only a few years ago about the work at Harwell, Weekes stated: “The account of these elegant experiments is a must-read for all newcomers to the field” (Weekes 2006). He also summed up well that first experiment by Galbraith and Jelley: “It is not often that a new phenomenon can be discovered with such simple equipment and in such a short time, but it may also be true that it is not often that one finds experimental physicists with this adventurous spirit!”