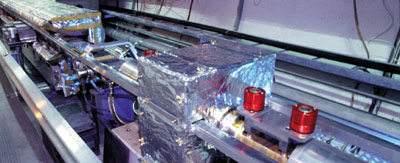
CERN’s LEP electronpositron collider has underlined the importance of precision measurements in particle physics. For this work it is vitally important to know the beam energy of this huge machine as accurately as possible.
In 1983, the UA1 and UA2 teams at CERN’s protonantiproton collider saw the Z particle the electrically neutral carrier of the weak interaction via a handful of events. By the mid-1990s the four experimental teams at CERN’s LEP electronpositron collider had accumulated millions of Zs.
The object of this massive data sample was to establish the consistency of the underlying Standard Model of particle physics. Before the sixth “top” quark was found at Fermilab’s Tevatron protonantiproton collider in 1994, this LEP data provided a valuable indication of where the top quark then the missing element of the Standard Model should lie.

With the discovery of the top quark, and the underlying Standard Model of physics in such good shape, the central requirement now is to pin down the parameters of the long-sought Higgs particle, which is responsible for the symmetry breaking at the heart of the Standard Model. Precision measurements could also aid the search for discrepancies signalling new physics effects beyond the Standard Model.
This “precision physics” continued as LEP moved away from its initial target at the Z resonance and moved to higher energies to explore the production of the W, the electrically charged partner of the Z, where a precision fix of the W mass could serve as a valuable benchmark. Before LEP went to higher energies, W mass information came via protonantiproton collision physics.
The first LEP W mass measurements in 1996 relied on determining the production threshold, but, as LEP energies were increased further, the W mass was determined by the kinematics of the production processes.
This need for precision has driven a continual need to measure the energy of the particle in LEP as accurately as possible, hopefully to within 10-20 MeV, or about 1 part in 10 000.
Thus began a programme to identify and compensate for external factors that could influence the beam energy measurement. Tiny tidal effects in LEP, amplified by the acceleration process, can contribute up to 40 MeV to the beam energy. Gravitational effects, owing to the neighbouring Jura mountains and the level of water in Lake Geneva, have to be allowed for. LEP energy calibration has even become an expensive way of monitoring the passage of French TGV high-speed trains.
The main technique for accurately measuring LEP beam energy has been resonant depolarization, in which the spin alignment of the stored electrons is destroyed. However, this is only precise at lower energies, around 60 GeV per beam. With LEP now approaching 100 GeV per beam, the low energy measurements have to be extrapolated over a long distance, limiting the attainable precision.
To sidestep this, LEP embarked on a project to mount a magnetic spectrometer inside the ring that will measure the beam energy directly via magnetic deviation.
The spectrometer is a steel dipole magnet, mounted in the LEP ring between the standard dipole magnets, with steel laminations in concrete, which are used to guide LEP’s particles. Beam position monitors either side of the spectrometer dipole track LEP particles as they enter and leave, the objective being to measure variations in the bending of the particles to an accuracy of 10-4. The adjacent monitors measuring to within 1 µm.
As LEP’s particles are bent round the ring, they continually emit a “screech” of synchrotron radiation that can reach levels of almost 1 kW/m. This emission is not dangerous in an uninhabited ring and disappears as soon as the machine is switched off. During operation, however, this radiation can heat up mechanical supports, producing expansions and contractions of the order of microns, which would mask the spectrometer measurements. To avoid this, the monitors are shielded by copper absorbers to soak up the synchrotron radiation. A stretched wire positioning system checks the relative position of the beam position monitors to within a 1 µm.
The system is now installed and recording preliminary data prior to regular operation later this year. The new spectrometer provides a vivid example of precision microengineering and electronic read-out for physics.