A new technique for cooling neutrons has been pioneered in Japan and offers the promise of an intense source of ultracold neutrons.
Physicists working at the spallation neutron source at the Research Centre for Nuclear Physics at Osaka University in Japan have for the first time produced ultracold neutrons using phonon excitations in a quantum liquid. A group led by Yasuhiro Masuda of KEK succeeded in the efficient production of ultracold neutrons in superfluid helium, which is free from the limitations of previous ultracold neutrons sources, imposed by Liouville’s theorem.
Ultracold neutrons (UCN) are important experimentally because, although neutrons are very small when compared with the interatomic distances in a material, UCN can be confined in a material bottle due to their wave properties. The attractive nuclear force inside a nucleus in a material distorts the wave associated with a neutron, pushing it back from the centre of the nucleus. Moreover, neutrons of long wavelength (low energy) see the nuclear force of many nuclei in a material. As a result, neutrons below a critical energy – UCN – are completely reflected from a material surface and can be confined in a bottle. UCN are also confined by the magnetic potential in a magnetic bottle (figure 1).
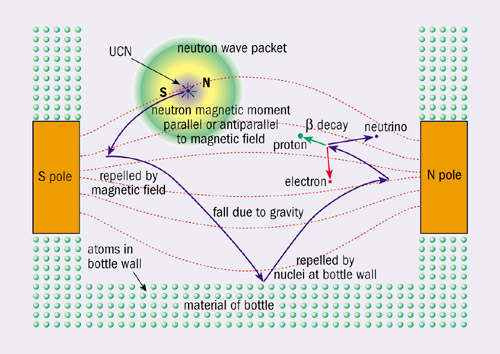
As neutrons are a fundamental constituent of the universe, confined neutrons can be used in various experiments to study the creation of matter in the universe, nucleosynthesis after the Big Bang, and the burning of the Sun. The energy available at the time of the Big Bang created a huge number of particle and antiparticle pairs, which annihilated and transformed back to energy. However, a CP-violating interaction broke the balance of particle and antiparticle numbers, and in due course quarks and leptons were formed. The quarks then condensed into protons and neutrons, and the protons and neutrons formed the nuclei of heavier elements in the process of nucleosynthesis. The nuclei later joined with electrons to form atoms, and eventually stars were born.
The neutron lifetime and the neutron cross-sections of nuclei together played a crucial role in nucleosynthesis immediately after the Big Bang. The neutron lifetime is also relevant to the proton-proton chain in the burning of the Sun. In addition, the same CP violating that created the imbalance between matter and antimatter in the early universe induces an electric dipole moment (EDM) in the neutron. UCN are used for precision measurements of both the EDM and the lifetime of the neutron, and can be used in neutron cross-section measurements. They are also useful for other precision experiments on neutron beta-decay and gravity, and are used in research in surface physics.
In any of these experiments, a high UCN density is very desirable. At the reactor at the Institut Laue-Langevin (ILL) in Grenoble, France, UCN have been extracted from a cold neutron source using gravity and a mechanical decelerator to produce the world’s highest UCN density – 10 UCN per cubic centimetre in an experimental bottle. Further improvement in the density is, however, not expected because of the limitations imposed by Liouville’s theorem, which says that the density in phase space should remain constant.
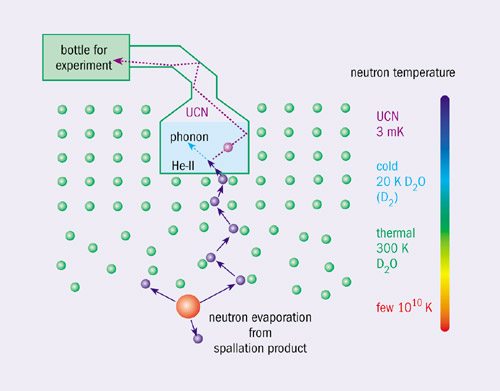
Now the Japanese group has employed a new UCN production method. Neutrons are produced in a spallation reaction, which generates a smaller photon (γ) to neutron production ratio than in a reactor. A pulsed proton beam, with a typical pulse width of 40 s and a power of 78 W, was used for the spallation reaction. The spallation neutrons, with energies in the MeV region, were then moderated down to cold neutron energies by collisions in thermal (300 K) and cold (20 K) heavy water (figure 2). The cold neutrons were further cooled down to UCN velocities through phonon interactions in 1.2 K superfluid helium. This cooling process is not limited by Liouville’s theorem because the decrease of neutron phase space is compensated by the increase in phase space of the phonons.
The UCN were then extracted, with negligible losses, through a guide tube into an experimental bottle, where the number of UCN was counted using two (15 and 24 mm diameter) solid-state detectors behind a 6Li film. A typical UCN count time was 60 s, and the UCN were found to remain in the bottle with a decay time constant of 14 s (figure 3). The UCN density was 0.7 UCN per cubic centimetre at the beginning of the counting, and this doubled to 1.4 UCN per cubic centimetre when the proton beam power was doubled.
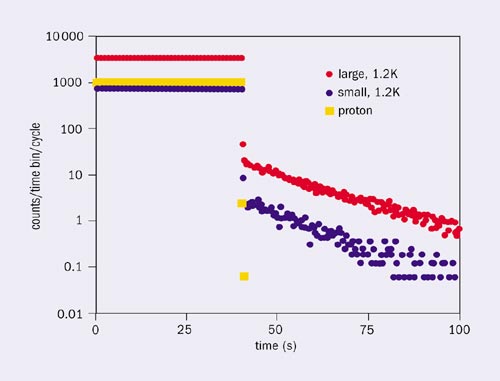
The new UCN source is expected to produce a UCN density of greater than 10,000 UCN per cubic centimetre, through improvements in the proton beam power, the UCN lifetime in the bottle, etc. The main limitation comes from the ability of the cryostat cooling to remove γ heating in the superfluid helium after the spallation reaction. The above expectation is based on practical values of superfluid helium temperature (0.8 K) and proton beam power (30 kW).
Further reading
Y Masuda et al. 2002 Phys. Rev. Lett. 89 284801.