EPAC returned to Italy for the last meeting in the European series.

Image credit: all photos courtesy Claudio Federici from LNF/INFN.
The European Particle Accelerator Conference (EPAC) has been a regular feature on the conference calendar since the first meeting in Rome 20 years ago. It brings together accelerator specialists working in areas ranging from fundamental physics through applications in material science, biology and medicine to commercialization in industry. EPAC ’08, the 11th in the series, took place in Genoa, the Italian port city with links to Christopher Columbus and Marco Polo – explorers who established some of the first routes from Europe to the Americas and the Far East. It was a fitting location, because this was the final conference in the series. EPAC will merge with the related American and Asian conferences into the International Particle Accelerator Conference (IPAC), which will roam between America, Asia and Europe on a three-yearly basis.
High energies and high intensities continue to be the major goals in the field, with a common thread of superconducting technology. The drive for high energies comes principally from high-energy particle physics, where CERN’s LHC is poised to lead the field. The machine was entering the final stages of hardware commissioning at the time of the conference. It is often pointed out that the LHC is its own prototype, breaking new ground in many ways in terms of scale and complexity – from the world’s largest vacuum system to the quench-protection systems and interlocks. Problems have been inevitable; the latest (Mobilizing for the LHC) will surely be overcome as previous ones were. One lesson that can be learned for future projects of this scale is not to forget the infrastructure while focusing on the more challenging aspects.
High aspirations
For now, the Tevatron at Fermilab and RHIC at Brookhaven continue to provide the high-energy frontier in hadron collisions. Thanks to a number of improvements, the Tevatron reached a peak luminosity of 3.15 × 1032 cm–2s–1 in 2008, exceeding the goals of the upgrade for Run II. Beam–beam interactions remain a major limitation, but work on compensation using two electron lenses installed in the ring is providing promising results in increasing the lifetime of the proton beam. Similarly RHIC has exceeded its design parameters, not only with gold–gold collisions but also in operating as a high-luminosity polarized-proton collider. Runs have reached a peak luminosity of about 35 × 1030 cm–2s–1 and a polarization of around 60% for a 100 GeV proton beam. To reach higher luminosities, electron cooling of the heavy-ion beams is being investigated, and a 20 MeV energy-recovery linac (ERL) is under construction for tests. This could also be used to study the new idea of coherent electron cooling in which density variations induced in the electron beam by the hadron beam are amplified by a free-electron laser (FEL) and fed back to correct the hadron beam – a variation on stochastic cooling. In addition, the ERL can test design ideas for the proposed electron–ion collider, e-RHIC.

For some years the international particle-physics community has been pursuing options for a future linear electron–positron collider to complement the high-energy hadron collisions at the LHC. In 2004 the International Technology Recommendation Panel decided that a future International Linear Collider operating in the 0.5 TeV centre-of-mass region should be based on 1.3 GHz superconducting RF technology. The Reference Design Report released in 2007 specified two 11 km linacs with an accelerating gradient of 31.5 MV/m and a peak luminosity of 2 × 1034 cm–2s–1.
A major goal of the first stage of the technical-design phase is to demonstrate by mid-2010 the feasibility of an accelerating gradient of 35 MV/m in 9-cell cavities, thereby allowing an operating margin of 10%. Global R&D on cavity shapes, fabrication and surface preparation is under way to meet this challenge. While tests have achieved field gradients as high as 41 MV/m, average results worldwide are still 15–20% short of reliably meeting the design requirement. A further challenge is the beam-delivery system, and particularly the issue of chromaticity, which is being investigated by a large international collaboration, with dedicated test facilities existing and under construction at SLAC and at KEK. The positron source is another challenging area because the design luminosity demands a source that delivers 1000 times as many particles per pulse than previous sources, together with the added complication of a possible upgrade to high (60%) polarization. Tests at SLAC have shown that a superconducting-undulator solution is feasible and development work is now in progress in the UK.
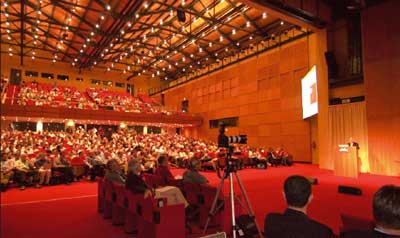
In parallel, an increasing international effort in the Compact Linear Collider (CLIC) study is investigating an innovative two-beam accelerator concept, which aims at a centre-of-mass energy of 3 TeV and a luminosity of 2 × 1034 cm–2s–1. Tests are under way at CERN in the CLIC Test Facility with a view to the production of a conceptual design report by 2010. Accelerating structures have already been tested to the required field gradient of 100 MV/m.
More futuristic is the attempt to harness electric fields in plasmas to accelerate particles (e.g. in the plasma-wakefield approach). Studies at the Accelerator Test Facility at Brookhaven are using multiple electron bunches only 5.5 ps long to generate the wakefields in a potentially more efficient manner. New results demonstrate a maximum wakefield of around 22 MV/m near the tail of the bunched beam. At Lawrence Berkeley National Laboratory, tests with laser wakefields reached 1 GeV in a distance of only 3 m in 2006. The goal now is to develop this principle into a laser accelerator to drive a short-wavelength FEL.
Current plans for the LHC foresee a series of luminosity upgrades by 2017, with a new injection system that could easily be upgraded to provide a multimegawatt beam. Another future option, which is still in the embryonic stage, would be to build an electron ring in the LHC tunnel to allow electron–proton collisions. The aim is for a luminosity of 1.1 × 1033 cm–2s–1, some 10 times as high as at DESY’s HERA collider, which ceased operations in 2007. Another option would be for a 140 GeV linac to supply the electrons. In either case, ERL technology should prove useful.
Intense and brilliant
In other areas of particle physics, the emphasis is on high intensities, because physics beyond the Standard Model may well lie in processes that are rare and/or difficult to observe. By the time it ceased operations in April 2008, the PEP-II B factory at SLAC had surpassed its design luminosity by a factor of four, reaching 1.2 × 1034 cm–2s–1, thanks to successive improvements, including continuous injection. The machine also provided valuable lessons to be learned for the design of future machines, such as in feedback. Elsewhere, commissioning is in full swing on the upgrade of the Beijing Electron–Positron Collider, BEPCII, to increase the collision rate by a factor of 100. At the Budker Institute of Nuclear Physics in Novosibirsk the aim is to achieve higher luminosity in the VEPP-2000 collider by means of innovative ideas, using existing injectors in a restricted area.
KEKB, the asymmetric e+e– collider for the study of B mesons at KEK, has operated with crab cavities for more than a year, the first installation to do so, following 13 years of R&D. The scheme using a single cavity per ring gives somewhat lower luminosity than without, but also at a lower beam current. The system needs further study to understand the effects limiting the luminosity at higher currents. A similar R&D programme at the DAΦNE e+e– collider at Frascati is investigating the use of a “crab waist” scheme, with sextupoles that give a factor of three shrinkage in the vertical plane as well as a narrower crossing. Put into operation in early 2008, they reached a peak luminosity 50% higher than the previous record, but at lower currents and two-thirds power.
Elsewhere, high-intensity facilities offer the possibility of a range of research. At the Japan Proton Accelerator Complex (J-PARC), the main ring is a 50 GeV synchrotron designed to deliver a 0.75 MW beam. This will serve kaon-rare decay studies, for example, and provide the first-ever megawatt-class fast-extracted beam to create neutrinos for the Tokai to Kamioka experiment. It will be fed by a 3 GeV 1 MW rapid-cycling synchrotron (RCS), which will also serve muon and neutron production targets in the Materials and Life Science Facility. The RCS already operates at design energy in conditions corresponding to a beam power of about 130 kW.
The Facility for Antiproton and Ion Research (FAIR), which is planned for Darmstadt, is set to be the largest science project funded in Europe in the next decade. It will provide beams of antiprotons and heavy ions at intensities 100 times as great as present, with additional capabilities for fragment-separation and plasma physics using ion bunches and petawatt lasers. Technical challenges surround the high-current beams, control of the dynamic-vacuum pressure and the design of rapid-cycling superconducting magnets. With two linacs and eight rings (including four storage rings), the FAIR complex will involve interesting beam manipulations with implications for RF synchronization. Experiments are expected in 2013.
The techniques of in-flight separation and isotope separation online (ISOL) are currently providing a turning point for research using radioactive beams. Superconducting linacs are the key in providing beams of heavy ions, while cyclotrons and synchrotrons are needed to reach high energies at in-flight facilities such as FAIR and the Radioisotope Beam Factory at the RIKEN institute in Japan. Studies in Europe, meanwhile, are leading towards the European ISOL facility, EURISOL, with a planned beam power of 5 MW. In the US both the National Superconducting Cyclotron Laboratory at Michigan State University and the Argonne National Laboratory have proposals for facilities that include the possibility of reaccelerating rare isotopes. TRIUMF has a slightly different proposal to use a megawatt-class electron linac for the photofission of a uranium target to produce neutron-rich rare isotopes (MW linacs could supply medical isotopes).
Design ideas for the necessary low-energy deuteron accelerator are already being pursued in the context of an international agreement between Euratom and Japan
Neutron sources also demand high-incident beam intensities for neutron production. The Spallation Neutron Source at Oak Ridge National Laboratory has a design-beam power of 1.4 MW, which is to be achieved after three years of operation. Since start-up in October 2006 the facility has reached 0.52 MW, making it the world’s most powerful spallation neutron source. There have been problems, however, with the low-energy beam transport and the superconducting 1 GeV proton linac. An intense neutron source is also a key element of the International Fusion Materials Irradiation Facility, which will study the responses of materials to the high flux of neutrons (1018 n/m2s) that would be emitted in a future fusion reactor. Design ideas for the necessary low-energy deuteron accelerator are already being pursued in the context of an international agreement between Euratom and Japan.
For facilities using FELs to provide short-wavelength photon beams for a variety of science, the key word is “brilliance”. While synchrotron sources can provide high energies (and hence short wavelengths), FELs offer the route to increased brilliance. The Free-electron Laser in Hamburg (FLASH) at DESY has been operating successfully for more than a year, delivering pulses at 6.5 nm. For the future, there is ongoing accelerator R&D for an X-ray FEL (XFEL) based on a 17.5 GeV electron linac, compared with the 1 GeV linac in FLASH. At SLAC, meanwhile, the Linac Coherent Light Source is under construction to operate at X-ray wavelengths (0.15–1.5 nm). This will use a new 135 MeV injector and the downstream third of the famous 3 km linac to reach a final energy of 14 GeV. It is on course to provide X-rays for the first experiments in summer 2009.
Beyond the laboratory
The main use of particle accelerators is outside research, particularly in X-ray machines in medicine. Now an increasing number of machines are being designed and built explicitly for hadron therapy using protons and carbon ions. In April 2007, PSI started the treatment of patients with a proton-scanning system based on a commercially supplied 250 MeV superconducting cyclotron. The Heidelberg Ion Therapy Facility is set to be Europe’s first dedicated proton- and carbon-therapy facility, with a synchrotron to provide the particles. Commissioning for three fixed beams was finished in April 2008, and commissioning of the gantry for scanning has begun. In Italy, the Centro Nazionale di Adroterapia Oncologica in Pavia is under construction, and commissioning the sources and the low-energy beam transfer is also under way.
As hadron therapy moves out of research laboratories and into hospitals, there is a growing market that industry can serve by providing not only the basic accelerators but also other items and services related to the treatment of patients. In this area, as well as in others, collaborations between industry and researchers provide an important means of transferring from a project to a product.
Indeed, working closely with industry is an increasingly important part of the accelerator scene. The LHC has led the way for “mega projects”, with industrialization of the magnet construction. One problem, however, is that the duration of such big projects can be longer than the lifetime of some companies. Nevertheless, co-operation with industry is essential from an early stage. The European XFEL project has already begun to work with industry in the production of the 100 cryomodules – each with eight 9-cell superconducting RF cavities and superconducting magnets. At the same time, research institutes that require linacs can benefit from being able to acquire customized systems direct from industry, particularly from the company ACCEL Instruments. There are many other specialized areas where partnerships between research and industry have proved mutually beneficial.
The accelerator scene continues to evolve and grow, not only in underlying technology, but also in its relations with other areas of science and industry. It is therefore fitting that the conference scene should reflect this evolution. The last EPAC provided a worthy ending to a successful series and the community now looks forward to the first IPAC, in 2010 in Kyoto, following the last in the Particle Accelerator Conference series, in Vancouver in May this year.
• The organizing committee for EPAC ’08, chaired by Caterina Biscari of INFN, and the scientific programme committee, chaired by Oliver Bruning of CERN, were formed by the board elected by the European Physical Society Accelerator Group, plus representatives of APAC and PAC, while the local organizing committee, chaired by Paolo Pierini of INFN, included a dozen members from INFN (Genoa, LASA, LNF), SINCROTRONE Trieste and CERN.
EPAC ’08: a hard act to follow
EPAC ’08 was a resounding success: the fruit of 20 years of experience in organizing these events with exciting scientific programmes reflecting the state of the art. More than 1000 delegates from 38 countries converged on EPAC ’08, the last in the biennial series as the conference joins Asia and North America to propose an International Particle Accelerator Conference on a three-year cycle.
As is customary for EPAC, the four-and-a-half-day programme consisted of plenary sessions at the opening, closing and prize sessions (with no more than two oral sessions in parallel), followed each afternoon by plenary poster sessions, allowing delegates to derive maximum benefit from the scientific programme. The meeting was augmented by the regular industrial exhibition, which for EPAC ’08 was the largest ever, with 90 companies participating. Many conference delegates also attended the traditional session for industry.
The 2008 prizes for the European Physical Society Accelerator Group were awarded during the prize ceremony, with the Rolf Wideröe prize going to Alex Chao of SLAC; the Gersh Budker prize to Norbert Holtkamp now at ITER and formerly of the Oak Ridge National Laboratory (ORNL) and Spallation Neutron Source (SNS); and the Frank Sacherer prize to Viatcheslav Danilov, also of ORNL/SNS.
With the continuing financial support of European laboratories, 66 students from around the world attended the conference. They had an extra opportunity to present their work in a special student-poster session. A prize was awarded in two categories: for a young physicist or engineer for quality of work and promise for the future; and for best posters. The prize for the best student poster went to Rocco Paparella of INFN–LASA.
Thanks to the team effort of the Joint Accelerator Conferences website (JACoW) collaboration editors, the proceedings were published “prepress” on the last day of the conference, and in record time at the JACoW open-access site three weeks later.