A new heavy-ion linear accelerator based in the US would provide the opportunity to tackle directly the current challenges arising from investigations of nuclear science.
Nuclear science is one of many branches of physics that daily disprove the musings of Lord Kelvin. Sometime around 1900, before quantum mechanics and special relativity, the pioneer of thermo-dynamics and the creator of the absolute-temperature scale reportedly declared, “There is nothing new to be discovered in physics now.” More than a century later, nuclear physicists remain energized by a host of pursuits, including exploring the science of atomic nuclei, understanding processes in nature’s most powerful explosion, the supernova, and addressing open questions about fundamental symmetries of nature. The compelling questions that drive this research are creating a push for new facilities in various parts of the world, including a rare-isotope science facility for the US.
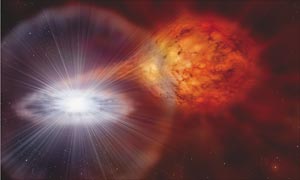
Rare-isotope research today explores the limits of nuclear stability and determines nuclear properties in the uncharted domain of nuclides with very unusual proton-to-neutron composition. The nuclei farthest from stability are especially important and provide the best vehicle for understanding the interplay of internal structure, reactions with other objects of a similar nature, and decays to the continuum. Such nuclei enable myriad experimental possibilities that collectively will advance and possibly even transform nuclear theory. Some of the most likely experiments will address wide-ranging themes in nuclear physics, ranging from magic numbers to dynamical symmetries to the limits of stability. Others will home in on specific measurements of nuclear-shell structure aimed at elucidating the most important degrees of freedom.
Attempts at fine-grained analysis of nuclear structure invariably lead to innovation in the tools that underpin the workaday world of nuclear physics. One contemporary example is intermediate-energy Coulomb excitation, which allows critical information to be extracted from experiments with beam intensities of only a few tens of thousands of atoms a day. Another example is the precision determination of nuclear binding energies with Penning traps. Current experimental frontiers include studies of nuclear sizes, wave functions, half-lives and decay modes of exotic nuclei.
Beyond the relevance to basic nuclear-structure physics, rare-isotope research is increasingly vibrant at its edges, where the field connects to other intellectual pursuits such as mesoscopic quantum systems – which can be averaged over many atomic-scale systems – and astrophysics. To a very good approximation, we can describe nuclei as self-sustaining finite droplets of a two-component – neutron and proton – Fermi-liquid, the detailed properties of which depend on the delicate interplay of the strong, electromagnetic and weak interactions.
Advances in computation techniques have allowed accurate microscopic calculations of the properties of very light (A < 16) nuclei. For heavier nuclei, full microscopic treatments rapidly become unfeasible and additional approximations must be introduced to solve the underlying many-body quantum problem. This is mesoscopic study, between microscopic and macroscopic. Many exotic nuclei are systems of marginal stability for which coupling to the continuum is important. They are “open” mesoscopic quantum systems in which interactions among finite numbers of particles can be described by effective forces. Describing the interplay of internal structure and external interactions is relevant to other research areas in physics, including information processing, quantum chaos, decoherence and phase transformations.
Understanding mesoscopic quantum systems is important to progress in nanotechnology and quantum computing, which are areas of high interest in condensed-matter physics and quantum optics. In nanotechnology, the basic quantum many-body problem raises fundamental issues about the design and engineering of artificial mesoscopic systems in which complexity emerges from the elementary interactions of a relatively small number of constituents. Nuclear science addresses similar questions, though at femtometre rather than nanometre scale.
Nuclear processes shape much of the visible universe – one reason why astrophysics and nuclear physics have long been closely connected. This link will strengthen in the future. Research with rare isotopes together with progress in observational astronomy will help address several areas of inquiry in astrophysics, including the chemical history of the universe, the conditions and sites where the elements were created, and the nature of exotic objects such as neutron stars, and explosive events such as novae and supernovae.

With more than 2000 active scientists worldwide, rare-isotope research is vibrant and international. New and planned facilities at RIKEN in Japan, GSI in Germany and GANIL in France complement existing facilities such as Louvain-la-Neuve in Belgium, HRIBF in Tennessee and the TRIUMF ISAC facility in Canada.
Inspired by such promise in this field, the US National Academies have recently published a report justifying the case for a new isotope science facility in the US. The report, nearly a year in the making and released online in unedited prepublication form on 8 December, concluded that the science goals were compelling and that “the science addressed by a rare-isotope science facility… should be a high priority for the United States”. The report adds that, provided the new facility is based on a heavy-ion linac, it will complement existing and planned nuclear-science activities worldwide.
A January town meeting in Chicago provided additional momentum. The aim of the meeting, part of the US nuclear-science community’s current five-year strategic planning exercise, was to identify top priorities in nuclear-structure and nuclear-astrophysics research. Attendees at the meeting concluded that one such priority is a more powerful means for producing rare isotopes for research with stopped, reaccelerated and in-flight (or fast) beams.
The scientific questions and a set of possible options for the technical implementation of such a new facility are laid out in some detail in a recent whitepaper released by the National Super-conducting Cyclotron Laboratory (NSCL) at Michigan State University (MSU). This document proposes building a high-power superconducting heavy-ion linac at MSU. The new Isotope Science Facility (ISF), the working name of the proposed MSU facility, would be based on a linac able to deliver beams of all stable elements with variable energies up to at least 200 MeV/nucleon and beam power up to 400 kW. A team at Argonne National Laboratory has presented similar ideas.
The ISF would combine the possibility of measurements with post-accelerated radioactive beams with the ability to conduct experiments using fast radioactive beams. In many cases, fast beams would provide 10,000 times higher sensitivity than is possible with reaccelerated beams, and make possible experiments with single ions of the rarest isotopes. This is an important consideration, the NSCL whitepaper points out, given that many interesting isotopes that are potential objects of study are produced at levels below a hundred or so per second, where the reaccelerated beam technique starts to become difficult. For example, fast beams would allow researchers to probe how nuclear structure evolves in nickel isotopes moving from atomic numbers 48 to 83. In addition fast beams would enable the study of key benchmark nuclei near 48Ni, 60Ca, 78Ni and 100Sn.

Complementary to the fast-beam approach, the proposed facility will allow isotope separation online (ISOL) techniques, in which isotopes are produced at rest in a thick target. CERN’s ISOLDE facility pioneered the field, and the forefront research using reaccelerated beam produced from ISOL continues in Geneva. Stopped beams are important for precision measurements with ion or atom traps or for collinear laser spectroscopy. Reaccelerated beams provide the opportunity to measure important nuclear-reaction rates relevant to nuclear astrophysics and to employ the well-proven techniques of nuclear-structure physics to a host of new nuclei. In addition, reaccelerated beams allow the investigation of fusion reactions, which will lead to the production of new neutron-rich isotopes of very heavy elements.
The use of a heavy-ion linac allows in-flight separation of ions and provides a path to reaccelerated beams that overcomes some of the chemical limitations of traditional ISOL techniques. Stopping, extracting and reaccelerating rare-isotope beams leads to intensity losses, the full extent of which is not yet known, although NSCL, Argonne National Laboratory, GSI and RIKEN are making significant progress. The time has come, however, for full performance tests of the concept. NSCL is building a project to test comprehensively the production, gas-stopping and reacceleration sequence.
A new US facility would complement international efforts in this field and would be relevant far beyond basic nuclear-structure research, especially given the links to other physics-related disciplines, such as astrophysics and mesoscopic science. However, the most important reason to proceed with rare-isotope research is to address questions at the core of nuclear physics. What are the limits of nuclear existence? How do we develop a predictive theory of nuclei? What is the origin of simple patterns in complex nuclei? What is the nature of neutron stars?
Such big questions represent the barest fraction of the unknown in nuclear science, which demonstrates that there is much compelling knowledge to be generated in a next-generation isotope-science facility – and also that Lord Kelvin is as wrong today as he was more than a century ago.
Further reading
The NSCL whitepaper, “Isotope Science Facility at Michigan State University,” is available at www.nscl.msu.edu/isf.
The National Academies Report, “Scientific Opportunities with a Rare-Isotope Facility in the United States,” is available at www7.nationalacademies.org/bpa/RISAC.html.