Physicists met in Uppsala to consider how the next generation of high-energy neutrino detectors can contribute to new physics as well as to study cosmic phenomena.

Neutrino telescopes are the biggest particle detectors. IceCube, currently being built at the South Pole, will have a 1 km3 instrumented volume when complete, and a similar project, KM3NET, is planned for the Mediterranean. Detectors such as AMANDA and the Baikal Neutrino Telescope have reached effective detection areas of tens of thousands of square metres. These huge arrays of photomultiplier tubes buried deep in clear ice or water primarily search the sky for high-energy neutrinos from violent cosmic phenomena, including gamma-ray bursts, active galactic nuclei and supernovae remnants. However, detecting extraterrestrial neutrinos can also provide a unique window on physics beyond the Standard Model of particle physics, the topics ranging from searches for new particles to the effects of extra dimensions.
On 20–22 September 2006 the Department of Nuclear and Particle Physics of Uppsala University hosted the first Workshop on Exotic Physics with Neutrino Telescopes. It focused on physics with neutrino telescopes, beyond astrophysics. The next generation of such detectors will be operational in less than a decade and will push the sensitivity of new physics to levels that can probe many existing theoretical models. At Uppsala we felt that it was timely to provide a forum to summarize the current status and where we can go in the next few years.
Research in underground labs or in accelerators is an important counterpart to searches using neutrino telescopes
Research in underground labs or in accelerators is an important counterpart to searches using neutrino telescopes. The first session reviewed accelerator results on new physics beyond the Standard Model in the post-LEP era, and discussed where the LHC will lead. It also summarized the results and perspectives of searches in underground labs. These searches complement each other, and the understanding of any new effect will need signals observed using different detection techniques to be coherently interpreted.
Searching for dark matter
There were also reviews from the smaller experiments such as MACRO, Super-Kamiokande or the Baksan Neutrino Observatory. During the 1990s, these collaborations provided the first limits on searches for new particles and dark matter, as well as on scenarios for new fundamental physics.
The search for dark-matter candidates is perhaps the most developed of the “exotic” topics covered by neutrino telescopes, both theoretically and experimentally. Particle physics provides several candidates for dark matter in the form of weakly interacting massive particles (WIMPs) that have survived from the Big Bang. The neutralino of the minimal supersymmetric Standard Model (MSSM) is one of them, but the lightest Kaluza–Klein mode, which arises in models with extra space–time dimensions, is also viable. If they exist, such particles should cluster gravitationally as halos in galaxies, and by the same principle accumulate in the centre of heavy objects, such as the Sun or the Earth. If the concentration is high enough, they could annihilate in pairs, producing neutrinos as a by-product. Neutrino telescopes are looking for an excess of neutrinos from the centre of the Sun or the Earth, which would indicate this process. There are competitive limits from the MACRO, Super-Kamiokande, Baksan, Baikal and AMANDA detectors, and experiments have begun to probe MSSM parameter space.
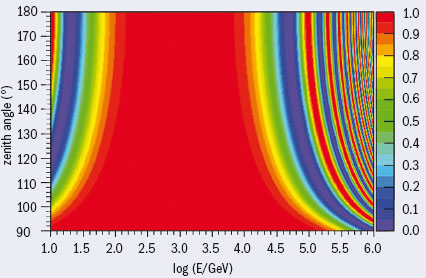
More exotic candidates of dark matter exist as non-topological solitons, or Q-balls. These are coherent stable states of quark, lepton and Higgs fields, and contrary to other WIMPS, they can be heavy, up to 100 TeV. Q-balls can leave a signature in a detector by catalysing proton decay as they pass through – the photomultiplier tubes of neutrino telescopes will record the Cherenkov light of the proton decay products. Another possibility is stable strange-quark matter in the form of nuclearites, with baryon numbers up to 1023, but low values of Z/A, the ratio of atomic number (Z) to atomic mass (A). Such particles could also explain cosmic rays above the Greisen–Zatsepin–Kuzmin (GZK) cut-off, if next-generation air-shower arrays confirm such high-energy particles.
Mini black holes and multi-bangs
The production of mini black holes in the collisions of high-energy neutrinos with the partons in matter nuclei is one manifestation of low-scale gravity. If the centre-of-mass energy of the interaction exceeds the Planck scale, a microscopic black hole can form in the interaction. However, in our 4D world, the Planck scale lies at energies of the Planck mass, around 1019 GeV, while the best man-made accelerators reach only tera-electron-volt energies (103 GeV) in the centre of mass. But in 4+D space–time dimensions the Planck scale may be much lower, and a 1010 GeV neutrino interacting with a nucleus inside the detector could produce a mini black hole. Although this might seem an extremely high energy, such neutrinos should be guaranteed by interactions of the flux of cosmic rays with the all-permeating cosmic-microwave relic photons.
A neutrino telescope could detect the immediate Hawking evaporation of a mini black hole in a burst of Standard Model particles (in around 10-27 s) through the emission of Cherenkov light by the products. There are many free parameters in models with extra dimensions and the uncertainties in the predictions are large. However, up to 10 black-hole events a year could be expected in a 1 km3 detector in the most favourable scenarios, taking into account the existing limits on the ultra-high-energy neutrino flux.
The gravity models at tera-electron-volt energies provide another intriguing possibility: elastic neutrino–parton scattering through the exchange of D-dimensional gravitons. Unlike in black-hole production, the neutrino is not destroyed, and continues on its way ready for another elastic interaction after a mean free path that, for a given energy, depends on the number of extra dimensions. The energy lost in each interaction goes into a hadronic shower, producing a very unusual signature in a neutrino telescope: multiple particle showers without a lepton among them. Current calculations predict that a 1 km3 detector could detect a handful of events each year, probing up to D = 6 extra dimensions.
Tests of fundamental physics
It is now eight years since Super-Kamiokande announced the observation of neutrino oscillations, and this effect continues to be the only established observation of physics beyond the Standard Model. We understand neutrino oscillations as a typical quantum-mechanical superposition effect between propagation (mass) and flavour states. However, there can be other causes of oscillations if certain fundamental physics laws are broken at some scale. These include violation of the equivalence principle (VEP), where the different neutrinos couple differently to the gravitational potential, violation of Lorentz invariance (VLI), where the different neutrinos can achieve different asymptotic velocities giving rise to velocity-induced oscillations, or non-standard neutrino interactions with matter at very high energies.
Fifty physicists from 16 countries attended the workshop
Results from Super-Kamiokande, MACRO and the Sudbury Neutrino Observatory show that, if they exist, such processes are subdominant, and there are limits on their relative strength. However, their dependence on the energy of the neutrino makes such processes interesting for large-scale neutrino telescopes. While the wavelength of standard oscillations is proportional to Eν, in the case of VEP or VLI the oscillation wavelength is proportional to 1/Eν, and neutrino telescopes will provide much better sensitivity, for example by looking for distortions of the angular dependence of the high-energy tail of the atmospheric neutrino flux.
Other contributions to the workshop covered the possibility of explaining trans-GZK cosmic rays as neutrinos with an increased interaction cross-section with matter at ultra-high energies; strongly interacting neutrinos; and how top-down scenarios can produce high-energy neutrinos from the decay products of super-massive Big Bang relics or topological defects. No doubt a discussion on vortons or monopolonia belongs to a workshop on exotic physics.
Fifty physicists from 16 countries attended the workshop. The Ångström laboratory, housing the Uppsala University physics departments and the newest building in one of the oldest universities in Europe, provided a pleasant venue for the meeting.
Further reading
All talks are available on the workshop Web page, which is at www.physics.uu.se/en/page/epnt06.