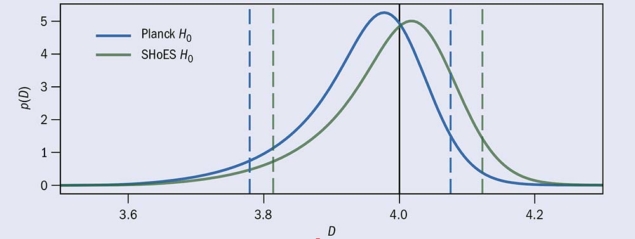
General relativity predicts very accurately how objects fall from a table and how planets move within the solar system. At larger scales, however, some issues arise. The most glaring is the theory’s prediction of the motion of stars within a galaxy and of the acceleration of galaxies away from each other, both of which are at odds with observations. Models containing dark matter and dark energy can solve these two problems, respectively. Another potential solution is that space–time contains additional dimensions, modifying general relativity. Such additional dimensions are not observable with electromagnetic waves, but new information gleaned from gravitational waves (GWs) are allowing such models to be tested for the first time.
Some modifications of general relativity, such as the Dvali–Gabadadze–Porrati (DGP) model, involve the addition of extra dimensions accessible to gravity. If such extra dimensions are large, and thus not rolled up to a microscopic size as predicted by some beyond-Standard Model theories, part of the gravitational field would “leak” into the extra dimensions. Therefore, GWs arriving at detectors such as those of the LIGO and VIRGO observatories would be weaker than expected.
The first GWs detected, in September 2015, came from distant black-hole binaries. For such objects, there is no electromagnetic-wave counterpart, so the only information astronomers have about their distance from Earth is from the GWs themselves, making it impossible to check if some of the wave’s intensity was lost. However, GW170817, the first observed merger of binary neutron stars, produced both GWs and electromagnetic radiation, which was measured by a wide range of instruments (CERN Courier December 2017 p16). As a result, we know in which galaxy the merger took place and therefore have a good measurement of the distance the GWs travelled. Using this distance measurement and the measured strength of the GW signal, one can test whether the signal follows general relativity or a model with additional dimensions.
Doing exactly this, a group led by Kris Pardo from Princeton University has found that the results are most compatible with the standard 3+1 space–time-dimensions picture. Assuming two values for the Hubble constant, as required due to a large discrepancy between values obtained by two different methods (CERN Courier May 2018 p17), the researchers show that, regardless of the value assumed, the results allow for a total of 4.0 ± 0.1 dimensions (see figure).
The authors also obtained an upper limit on the graviton’s lifetime of 450 million years. As is the case with a potential leakage of gravity into extra dimensions, the decay of gravitons propagating towards Earth would also cause the strength of the GW signal to decrease.
These findings are just the beginning of the physics studies made possible by gravitational-wave astronomy. As the authors make clear in their paper, the results only affect theories with finite but large-scale extra dimensions. That may change, however, as more GWs are expected to be measured, with increased precision, in the future. One promising parameter capable of probing a larger set of models is the polarisation of the GWs. For the GW170817 system, polarisation information was not available at the time of observation owing to the limited number of GW detectors. Any higher-dimensional model allows for extra GW polarisation modes, which can be studied with the help of additional GW detectors such as the planned KAGRA and IndIGO facilities.
With a future global array of GW detectors, we can look forward to more studies in this field of physics which, until now, has been almost inaccessible.
Further reading
K Pardo et al. 2018 J. Cosmol. Astropart. Phys. 7 48.