The Canfranc Underground Laboratory welcomes new ideas and proposals.
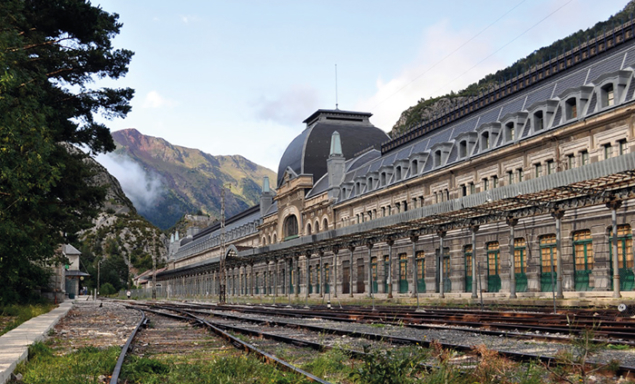
Image credit: LSC.
The Canfranc Underground Laboratory (LSC) in Spain is one of four European deep-underground laboratories, together with Gran Sasso (Italy), Modane (France) and Boulby (UK). The laboratory is located at Canfranc Estación, a small town in the Spanish Pyrenees situated about 1100 m above sea level. Canfranc is known for the railway tunnel that was inaugurated in 1928 to connect Spain and France. The huge station – 240 m long – was built on the Spanish side, and still stands as proof of the history of the place, although the railway operation was stopped in 1970.
In 1985, Angel Morales and his collaborators from the University of Zaragoza started to use the abandoned underground space to carry out astroparticle-physics experiments. In the beginning, the group used two service cavities, currently called LAB780. In 1994, during the excavation of the 8 km-long road tunnel (Somport tunnel), an experimental hall of 118 m2 was built 2520 m away from the Spanish entrance. This hall, called LAB2500, was used to install a number of experiments carried out by several international collaborations. In 2006, two additional larger halls – hall A and hall B, collectively called LAB2400 – were completed and ready for use. The LSC was born.
Today, some 8400 m3 are available to experimental installations at Canfranc in the main underground site (LAB2400), and a total volume of about 10,000 m3 on a surface area of 1600 m2 is available among the different underground structures. LAB2400 has about 850 m of rock overburden with a residual cosmic muon flux of about 4 × 10–3 m–2 s–1. The radiogenic neutron background (< 10 MeV) and the gamma-ray flux from natural radioactivity in the rock environment at the LSC are determined to be of the order of 3.5 × 10–6 n/(cm2 s) and 2γ/(cm2 s), respectively. The neutron flux is about 30 times less intense than on the surface. The radon level underground is kept in the order of 50–80 Bq/m3 by a ventilation system with fresh-air input of about 19,600 m3/h and 6300 m3/h for hall A and B, respectively. To reduce the natural levels of radioactivity, a new radon filtering system and a radon detector with a sensitivity of mBq/m3 will be installed in hall A in 2016, to be used by the experiments.
The underground infrastructure also includes a clean room to support detector assembly and to maintain the high level of cleanliness required for the most important components. A low-background screening facility, equipped with seven high-purity germanium γ-spectrometers, is available to experiments that need to select components with low radioactivity for their detectors. The screening facility has recently been used by the SuperKGd collaboration to measure the radiopurity of gadolinium salts for the Super-Kamiokande gadolinium project.

Image credit: LSC.
A network of 18 optical fibres, each equipped with humidity and temperature sensors, is installed in the main halls to monitor the rock stability. The sensitivity of the measurement is at the micrometer level; so far, across a timescale of four years, changes of 0.02% have been measured over 10 m scale lengths.
The underground infrastructure is complemented by a modern 1800 m2 building on the surface, which houses offices, a chemistry and an electronics laboratory, a workshop and a warehouse. Currently, some 280 scientists from around the world use the laboratory’s facilities to carry out their research.
The scientific programme at the LSC focuses on searches for dark matter and neutrinoless double beta decay, but it also includes experiments on geodynamics and on life in extreme environments.
Neutrinoless double beta decay
Unlike the two-neutrino mode observed in a number of nuclear decays (ββ2ν, e.g. 136Xe → 136Ba + 2e– + 2–νe), the neutrinoless mode of double beta decay (ββ0ν, e.g. 136Xe → 136Ba + 2e–) is as yet unobserved. The experimental signature of a neutrinoless double beta decay would be two electrons with total energy equal to the energy released in the nuclear transition. Observing this phenomenon would demonstrate that the neutrino is its own antiparticle, and is one of the main challenges in physics research carried out in underground laboratories. The NEXT experiment at the LSC aims to search for those experimental signatures in a high-pressure time projection chamber (TPC), using xenon enriched in 136Xe. The NEXT TPC is designed with a plane of photomultipliers on the cathode and a plane of silicon photomultipliers behind the anode. This set-up allows the collaboration to determine the energy and the topology of the event, respectively. In this way, background from natural radioactivity and from the environment can be accurately rejected. In its final configuration, NEXT will use 100 kg of 136Xe and 15 bar pressure. A demonstrator of the TPC with 10 kg of Xe, named NEW, is currently being commissioned at the LSC.

Image credit: LSC.
The Canfranc Laboratory also hosts R&D programmes in support of projects that will be carried out in other laboratories. An example is BiPo, a high-sensitivity facility that measures the radioactivity on thin foils for planar detectors. Currently, BiPo is performing measurements for the SuperNEMO project proposed at the Modane laboratory. SuperNEMO aims to make use of 100 kg of 82Se in thin foils to search for ββ0ν signatures. These foils must have very low contamination from other radioactive elements. In particular, the contamination must be less than 10 μBq/kg for 214Bi from the 238U decay chain, and less than 2 μBq/kg for 208Tl from the 232Th decay chain. These levels of radioactivity are too small to be measured with standard instruments. The BiPo experiment provides a technical solution to perform this very accurate measurement using a thin 82Se foil (40 mg/cm2) that is inserted between two detection modules equipped with scintillators and photomultipliers to tag 214Bi and 208Tl.
Dark matter
The direct detection of dark matter is another typical research activity of underground laboratories. At the LSC, two projects are in operation for this purpose: ANAIS and ArDM. In its final configuration, ANAIS will be an array of 20 ultrapure NaI(Tl) crystals aiming at investigating the annual modulation signature of dark-matter particles coming from the galactic halo. Each 12.5 kg crystal is put inside a high-purity electroformed copper shielding made at the LSC chemistry laboratory. Roman lead of 10 cm thickness, plus other lead structures totalling 20 cm thickness, are installed around the crystals, together with an active muon veto and passive neutron shielding. In 2016, the ANAIS detector will be in operation with a total of 112 kg high-purity NaI(Tl) crystals.
A different experimental approach is adopted by the ArDM detector. ArDM makes use of two tonnes of liquid argon to search for WIMP interactions in a two-phase TPC. The TPC is viewed by two arrays of 12 PMTs and can operate in single phase (liquid only) or double phase (liquid and gas). The single-phase operation mode was successfully tested up to summer 2015, and the collaboration will be starting the two-phase mode by the end of 2015.
Nuclear astrophysics
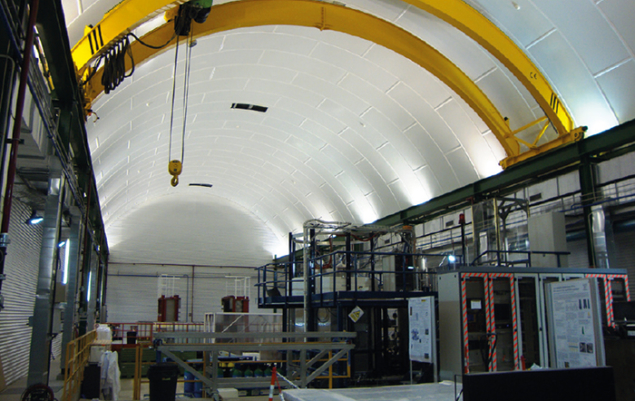
Image credit: LSC.
In recent decades, the scientific community has shown growing interest in measuring cross-sections of nuclear interactions taking place in stars. At the energy of interest (that is, the average energy of particles at the centre of the stars), the expected interaction rates are very small. The signal is so small that the measurement can only be performed in underground laboratories where the levels of background are reduced. For this reason, a project has been proposed at the LSC: the Canfranc Underground Nuclear Astrophysics (CUNA) facility. CUNA would require a new experimental hall to host a linear accelerator and the detectors. A feasibility study has been carried out and further developments are expected in the coming years.
Geodynamics
The geodynamic facility at the LSC aims to study local and global geodynamic events. The installation consists of a broadband seismometer, an accelerometer and two laser strainmeters underground, and two GPS stations on the surface in the surroundings of the underground laboratory. This facility allows seismic events to be studied over a wide spectrum, from seismic waves to tectonic deformations. The laser interferometer consists of two orthogonal 70 m-long strainmeters. Non-linear shallow water tides have been observed with this set-up and compared with predictions. This was possible because of the excellent signal-to-noise ratio for strain data at the LSC.
Life in extreme environments
In the 1990s, it became evident that life on Earth extends into the deep subsurface and extreme environments. Underground facilities can be an ideal laboratory for scientists specialising in astrobiology, environmental microbiology or other similar disciplines. The GOLLUM project proposed at the LSC aims to study micro-organisms inhabiting rocks underground. The project plans to sample the rock throughout the length of the railway tunnel and characterize microbial communities living at different depths (metagenomics) by DNA extraction.
Currently operating mainly in the field of dark matter and the search for rare decays, the LSC has the potential to grow as a multidisciplinary underground research infrastructure. Its large infrastructure equipped with specialized facilities allows the laboratory to host a variety of experimental projects. For example, the space previously used by the ROSEBUD experiment is now available to collaborations active in the field of direct dark-matter searches or exotic phenomena using scintillating bolometers or low-temperature detectors. A hut with exceptionally low acoustic and vibrational background, equipped with a 3 × 3 × 4.8 m3 Faraday cage, is available in hall B. This is a unique piece of equipment in an underground facility that, among other things, could be used to characterize new detectors for low-mass dark-matter particles. Moreover, some 100 m2 are currently unused in hall A. New ideas and proposals are welcome, and will be evaluated by the LSC International Scientific Committee.
• For further details about the LSC, visit www.lsc-canfranc.es.