by Per F Dahl, IOP Publishing, ISBN 0750308656, £55 ($75/€75).
Per Dahl continues to be a careful and conscientious compiler of physics history. After Flash of the Cathode Rays: A History of J J Thomson’s Electron (1997) and Heavy Water and the Wartime Race for Nuclear Energy (1999), his latest volume completes a trilogy published under the Institute of Physics imprint. The Heavy Water saga was compelling reading, but Nuclear Transmutation reverts to the solid scientific style of Flash of the Cathode Rays.
Nuclear Transmutation focuses on the competition between experimental teams in Europe and the US to furnish and exploit high-energy beams of subatomic particles in the quest to understand more about the atomic nucleus. Dahl, as in his other books, takes pains to place this central theme in a much broader context.
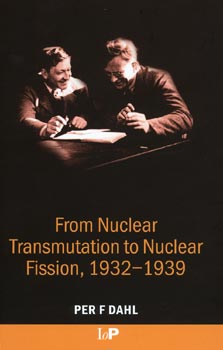
The period covered by the book links the twilight of the career of one physics giant named Ernest – Rutherford – with the emergence of another – Lawrence. The two main contenders in the competition were the Rutherford-inspired team of Cockcroft and Walton at Cambridge, and the group led by Lawrence at Berkeley. Their achievements and the subsequent award of the Nobel prize assured them a place in science history. Also prominent were the teams with Merle Tuve and Gregory Breit at the Carnegie Institution of Washington DC; Charles Lauritsen at Caltech; and Robert Van de Graaff at Princeton.
In the 1920s, the interior of the atomic nucleus was a complete mystery, but there was a widespread feeling that systematic studies using high-energy projectiles could reveal more about the nucleus. Each group developed its own technique to produce the necessary high voltages to accelerate projectile particles.
Many of Rutherford’s physics achievements came before he arrived at Cambridge in 1919. The road ahead was to be more difficult, demanding inspired teamwork, Rutherford’s dogged perseverance in his belief in the neutron, and the development and mastery of new techniques. However, Rutherford knew more than most about two complementary aspects of nuclear transformations: radioactive decay and reactions induced by radioactively emitted particles.
Rutherford’s era in Cambridge also coincided with the development of modern quantum mechanics. While the majority of quantum mechanics applications focused on atomic physics, a deeper implication was seen by George Gamow. Radioactive particles tunnel, rather than leap, out of the nucleus. Gamow soon realized that if this were the case, energetic particles could also tunnel their way in.
Gamow’s new ideas immediately raised the research temperature
Gordon Fraser
At Cambridge, Gamow’s new ideas immediately raised the research temperature. The payoff came in 1932 when Cockcroft and Walton “split the atom”. This, along with Chadwick’s discovery of the neutron the same year, was the high-water mark for Cambridge research, and soon the tide there began to ebb.
Dahl recounts how another tide rose in the US. In the mid-1920s, the young Lawrence had been dabbling in nondescript research. He and Tuve were both born in 1901 in the same small South Dakota town. Tuve, working under the astute Gregory Breit, wisely urged Lawrence to concentrate instead on digging deep in a potentially rich new physics vein – beams of subnuclear particles. Lawrence took the idea and ran with it, going on to invent the cyclotron. Dahl explains how Lawrence, obsessed with cyclotron performance, initially missed out on several major physics discoveries in the early 1930s. However, Lawrence’s powerful and versatile machines quickly overhauled the rest of the field, and he received the Nobel accolade in 1939, while recognition for Cockcroft and Walton’s pioneering achievement did not come until 1951. The lesson from Lawrence was that the development of big physics machines is not an end in itself, and must be complemented from the outset by physics insight and the provision of adequate detectors.
The history of accelerating subatomic particles has a curious Norwegian tradition. Both Lawrence and Tuve had Norwegian roots, as did Tuve’s collaborators Lawrence Hafstad and Odd Dahl (who in the early 1950s initially led the team that developed CERN’s first large accelerator). In Europe, accelerator pioneer Rolf Wideröe (whose work greatly influenced Lawrence) was also Norwegian. As Odd Dahl’s son, Per Dahl is well qualified to document this story.
Towards the end of the 1930s, the physics spotlight turned away from artificially induced reactions to nuclear fission, again a transatlantic affair and with many of the earlier figures continuing to play key roles. Fission, a different kind of physics, is a closing parenthesis in the book.
Dahl contends that the early history of experimental nuclear physics was a race. It was surely more complicated than that. The history of science and technology is full of examples of different approaches to a common goal, where success and failure is not a simple binary outcome. In the quest to study the deep structure of matter using precisely controlled conditions, the Cambridge researchers got off to a flying start because of their unrivalled expertise in studying radioactive decays and nuclear transmutations. However, it was Lawrence who went on to set the industrial standard.