The 10th meeting of the Quarkonium Working Group provides a good time to take stock.
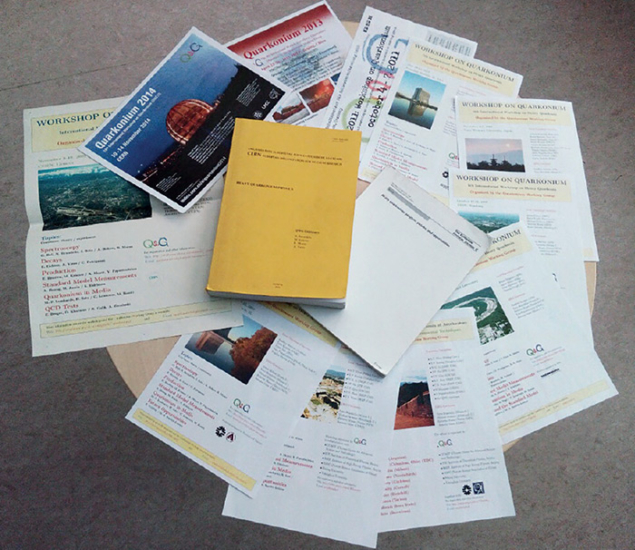
Image credit: A Vairo.
Quarkonium lies at the very foundation of quantum chromodynamics (QCD). In the 1970s, following the discovery of the J/ψ in 1974, the narrow width (and later the hyperfine splittings) of quarkonium states corroborated spectacularly asymptotic freedom as predicted by QCD in 1973 and served to establish it as the theory of the strong interaction (CERN Courier January/February 2013 p24). Further progress in explaining quarkonium physics in terms of QCD turned out, however, to be slow in coming and relied for a long time on models. The reason for these difficulties is that non-relativistic bound states, such as quarkonia, are multiscale systems. While some processes, such as annihilations, happen at the heavy-quark mass scale and, as a consequence of asymptotic freedom, are well described by perturbative QCD, all quarkonium observables are also affected by low-energy scales. If these scales are low enough for perturbative QCD to break down, then they call for a nonperturbative treatment.
In the 1990s, the development of non-relativistic effective field theories such as non-relativistic QCD (1986, 1995) and potential non-relativistic QCD (1997, 1999) led to a systematic factorization of high-energy effects from low-energy effects in quarkonia. Progress in lattice QCD allowed an accurate computation of the latter. Hence, the theory of quarkonium physics became fully connected to QCD. The founding of the Quarkonium Working Group (QWG) in 2002 was driven mostly by this theoretical progress and the urgency and enthusiasm to transmit the new paradigm. Electron–positron collider experiments (BaBar, Belle, BES, CLEO) and experiments at Fermilab’s Tevatron were yielding quarkonium data with unprecedented precision, and QCD was in a position to take full advantage of these data.
The QWG gathered together experimentalists and theorists to establish a common language, highlight unsolved problems, set future research directions, discuss the latest data, and suggest new analyses in quarkonium physics. Its first meeting took place at CERN in November 2002, where the urgency and enthusiasm of 2002 animated long evening sessions that eventually led to the first QWG document in 2005 (Quarkonium Working Group Collaboration 2005). This document reflects the original intent of the QWG: to rewrite quarkonium physics in the language of effective field theories, emphasizing its potential for systematic and precise QCD studies. But surprises were around the corner.
In 2003 the first observation of the X(3872) by Belle – which with more than 1000 citations is the most quoted result of the B-factories – opened an era of new spectroscopy studies sometimes called the “charmonium renaissance” (CERN Courier January/February 2004 p8). From 2003 onwards, several new states were found in the charmonium and bottomonium regions of the spectrum, and they were unlikely to be standard quarkonia. Some of them – the many charged states named Z±c and Z±b – surely were not. Suddenly quarkonium became again a tool for discoveries, not necessarily of new theories, but of new phenomena in the complex realm of low-energy QCD. The second QWG document in 2011 captured this overwhelming flow of new data and the surrounding excitement (Quarkonium Working Group Collaboration 2011). But more excitement and more new data were still to come.
Almost exactly 12 years after the first meeting organized by the QWG, quarkonium experts converged again on CERN for the group’s 10th meeting on 10–14 November 2014. Sponsored by the QWG, the 2014 meeting was organized locally by CERN affiliates and staff members, and supported by the LHC Physics Centre at CERN. The meeting began with several sessions devoted to spectroscopy, with the focus on the new spectroscopy. The ATLAS, Belle, BESIII, CMS and LHCb collaborations all presented new analyses and data. One surprise was BESIII’s observation of an e+e– → γX(3872) signal at s > 4 GeV, perhaps via Y(4260) → γX(3872). If confirmed it would relate two of the best known new states in the charmonium region and challenge the popular interpretation of the Y(4260) as a charmonium hybrid.

From the talk of X Garcia i Tormo.
In view of the many new states, theoretical effort has concentrated on finding a common framework that could describe them. Molecular interpretations, tetraquarks and threshold cusp-effects were among the possibilities discussed at the meeting. A novelty was the proposal to use lattice data to build hybrid and tetraquark multiplets within the Born–Oppenheimer approximation. Two lively round-table discussions debated the new states further. In a special discussion panel, the Particle Data Group members of the QWG asked for input in establishing a naming scheme for these states. The suggestion that eventually came from the QWG was to call the new states in the charmonium region XcJ if JPC= J++, YcJ if JPC = J–, PcJ if JPC = J–+ and ZcJ if JPC = J+–, and to follow a similar scheme in the bottomonium region.
Presenting new results
Non-relativistic effective field theories, perturbative QCD and lattice calculations played a major role in the sessions that were devoted to precise determinations of the heavy-quark masses, the strong coupling constant and other short-range quantities. Typical results required calculations with three-loop or higher accuracies. New results were presented on the leptonic width of the Υ(1S), the quarkonium spectrum, the heavy-quark masses, heavy-quark pair production at threshold and αs. Lattice QCD provided a valuable input in some of these determinations (figure 1) or an alternative derivation with comparable precision. On the experimental side, the KEDR collaboration highlighted some of its most recent precision measurements in the charmonium region below or close to threshold. Quarkonium observables may serve not only to constrain precisely Standard Model parameters in the QCD sector, but also to determine some otherwise difficult-to-access electroweak parameters. In particular, there was a report on the possibility of measuring the Hcc coupling in the radiative decay of the Higgs boson to J/ψ.
To isolate the relevant signal, it is important that the effects of cold nuclear matter are properly accounted for
The last two days of the workshop were devoted to quarkonium production at heavy-ion and hadron colliders. Measurements of quarkonium production cross-sections in heavy-ion and proton–heavy-ion collisions were presented by the LHC collaborations ALICE, CMS and LHCb, and by PHENIX and STAR at Brookhaven’s Relativistic Heavy-Ion Collider (RHIC). It has been known since 1986 that quarkonium dissociation – induced by the medium that is produced in heavy-ion collisions – may serve as a probe of the properties of the medium, possibly revealing the presence of a new state of matter.
To isolate the relevant signal, it is important that the effects of cold nuclear matter are properly accounted for. This is the motivation behind measurements of proton–heavy-ion collisions, and the many theoretical studies that were presented at the workshop. It is important to account for recombination effects and to look at tightly bound states that are less sensitive to nonperturbative contributions (bottomonium). It is also important to consider the dynamics of thermalization – a key ingredient to link spectral studies to actual data. Finally, it is important to have a controlled way to compute the underlying dissociation processes. This is where major progress was made in recent years with the development of non-relativistic effective field theories for quarkonium in a thermal bath. The main result has been a change in the understanding of quarkonium dissociation. Until recently, dissociation was mostly understood as a consequence of the screening that is induced by the medium, but, nowadays, additional mechanisms of dissociation have been identified, which under some circumstances may be more important than screening. Several speakers reported on the present theoretical situation, as well as lattice calculations in an effective-field-theory framework.
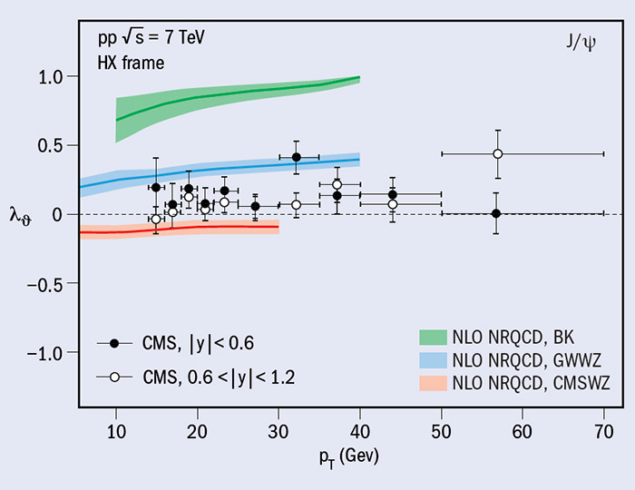
From the talks of G Bodwin and C Lourenço.
Quarkonium production mechanisms in hadron colliders are at the core of the modern understanding of quarkonium physics. The successful theoretical description of production data from the Tevatron through the so-called colour-octet mechanism helped to establish non-relativistic QCD as a suitable effective field theory for quarkonia in the 1990s. Predictions of non-relativistic QCD continue to be challenged by the enormous amount of data that has been provided over the past years by the experiments at DESY’s HERA collider and at the Tevatron and, most recently, by the LHC experiments. ALICE, ATLAS, CMS and LHCb all presented data on regions of large transverse-momentum that were, up to now, unexplored. The meeting discussed theoretical issues that arise in trying to describe these data, and emphasized the crucial role that experiments must play in resolving these issues. One such issue is that different determinations of the nonperturbative matrix elements of non-relativistic QCD, which rely on fitting to the data in different transverse-momentum regions and/or on different sets of observables, lead to different results. Some of these determinations fail to yield definite predictions for quarkonium polarizations, while others lead to polarization predictions that are in contradiction with polarization data (figure 2).
An important related issue is to establish clearly the transverse-momentum region in which non-relativistic QCD factorization holds. This issue is best addressed by having the greatest possible amount of cross-section and polarization data at high and low transverse-momenta for both charmonium and bottomonium states, including the P-wave χc and χb states. Some speakers pointed out that measurements of additional production processes may further constrain the non-relativistic QCD matrix elements. Finally, others suggested that a resolution of the theoretical issues may not be far away.
A celebration of quarkonium
Embedded in the workshop, Chris Quigg’s seminar in the CERN Physics Department’s series celebrated the first 40 years of quarkonium in the presence of many of the heroes of quarkonium physics. The talk, rich in anecdotes and insights, but also with many highlights on current directions, served as a delightful pause in the packed schedule of the workshop. It also served to put the workshop, whose discussion items focused on the advances of the past year and a half, on a broader, more historical perspective.
Quarkonium is a special system. Its multiscale nature with at least one large energy scale allows for systematic studies of QCD across a range of energy scales. Its clean experimental signatures have led over the years to a significant experimental programme, which is pursued today by, among others, the Belle and BES experiments as well as those at RHIC and at the LHC. Quarkonium has proved to be a competitive, sometimes unique, source for precise determinations of the parameters of the Standard Model (the strong-coupling constant, masses, Higgs-coupling to heavy quarks), a valuable probe for emergent QCD phenomena in vacuum (such as exotic bound states: hybrids, tetraquarks, molecules; or the colour-octet mechanism in quarkonium production and decay) and in medium (such as the state of matter formed in heavy-ion collisions); and, possibly, a probe for new physics. The QWG has provided during the past 12 years an organization in which the advance of quarkonium physics could be shared in a coherent framework among a wide community of physicists. The CERN workshop in 2014 was also a celebration of this achievement.