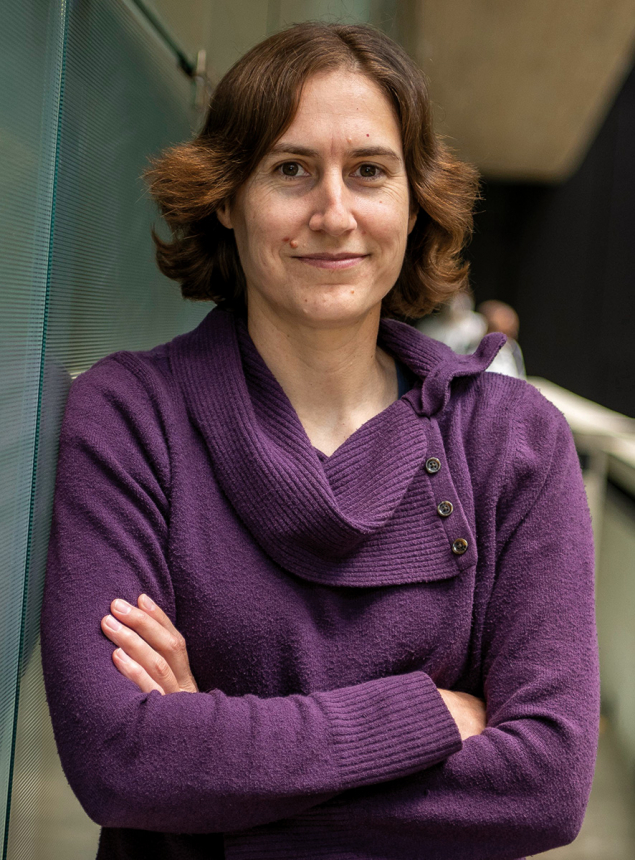
What role does science communication play in your academic career?
When I was a postdoc I started to realise that the science communication side of my life was really important to me. It felt like I was having a big impact – and in research, you don’t always feel like you’re having that big impact. When you’re a grad student or postdoc, you spend a lot of time dealing with rejection, feeling like you’re not making progress or you’re not good enough. I realised that with science communication, I was able to really feel like I did know something, and I was able to share that with people.
When I began to apply for faculty jobs, I realised I didn’t want to just do science writing as a nights and weekends job, I wanted it to be integrated into my career. Partially because I didn’t want to give up the opportunity to have that kind of impact, but also because I really enjoyed it. It was energising for me and helped me contextualise the work I was doing as a scientist.
How did you begin your career in science communication?
I’ve always enjoyed writing stories and poetry. At some point I figured out that I could write about science. When I went to grad school I took a class on science journalism and the professor helped me pitch some stories to magazines, and I started to do freelance science writing. Then I discovered Twitter. That was even better because I could share every little idea I had with a big audience. Between Twitter and freelance science writing, I garnered quite a large profile in science communication and that led to opportunities to speak and do more writing. At some point I was approached by agents and publishers about writing books.
Who is your audience?
When I’m not talking to other scientists, my main community is generally those who have a high-school education, but not necessarily a university education. I don’t tailor things to people who aren’t interested in science, or try to change people’s minds on whether science is a good idea. I try to help people who don’t have a science background feel empowered to learn about science. I think there are a lot of people who don’t see themselves as “science people”. I think that’s a silly concept but a lot of people conceptualise it that way. They feel like science is closed to them.
The more that science communicators can give people a moment of understanding, an insight into science, I think they can really help people get more involved in science. The best feedback I’ve ever gotten is when students have come up to me and said “I started studying physics because I followed you on Twitter and I saw that I could do this,” or they read my book and that inspired them. That’s absolutely the best thing that comes out of this. It is possible to have a big impact on individuals by doing social media and science communication – and hopefully change the situation in science itself over time.
What were your own preconceptions of academia?
I have been excited about science since I was a little kid. I saw that Stephen Hawking was called a cosmologist, so I decided I wanted to be a cosmologist too. I had this vision in my head that I would be a theoretical physicist. I thought that involved a lot of standing alone in a small room with a blackboard, writing equations and having eureka moments. That’s what was always depicted on TV: you just sit by yourself and think real hard. When I actually got into academia, I was surprised by how collaborative and social it is. That was probably the biggest difference between expectation and reality.
How do you communicate the challenges of academia, alongside the awe-inspiring discoveries and eureka moments?
I think it’s important to talk about what it’s really like to be an academic, in both good ways and bad. Most people outside of academia have no idea what we do, so it’s really valuable to share our experiences, both because it challenges stereotypes in terms of what we’re really motivated by and how we spend our time, but also because there are a lot of people who have the same impression I did: where you just sit alone in a room with a chalkboard. I believe it’s important to be clear about what you actually do in academia, so more people can see themselves happy in the job.
At the same time, there are challenges. Academia is hard and can be very isolating. My advice for early-career researchers is to have things other than science in your life. As a student you’re working on something that potentially no one else cares very much about, except maybe your supervisor. You’re going to be the world-expert on it for a while. It can be hard to go through that and not have anybody to talk to about your work. I think it’s important to acknowledge what people go through and encourage them to get support.
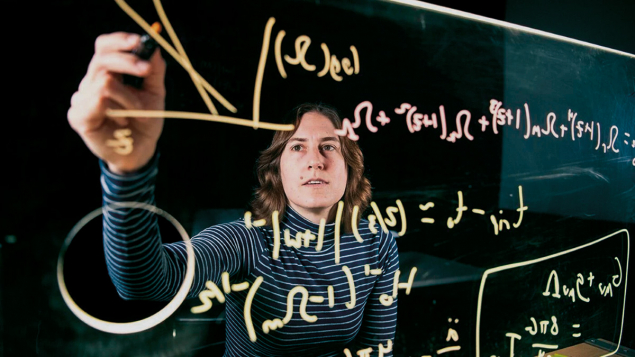
There are of course other parts of academia that can be really challenging, like moving all the time. I went from West coast to East coast between undergrad and grad school, and then from the US to the UK, from the UK to Australia, back to the US and then to Canada. That’s a lot. It’s hard. They’re all big moves so you lose whatever local support system you had and you have to start over in a new place, make new friends and get used to a whole new government bureaucracy.
So there are a whole lot of things that are difficult about academia, and you do need to acknowledge those because a lot of them affect equity. Some of these make it more challenging to have diversity in the field, and they disproportionately affect some groups more than others. It is important to talk about these issues instead of just sweeping people under the rug.
Do you think that social media can help to diversify science and research?
Yes! I think that a large reason why people from underrepresented groups leave science is because they lack the feeling of belonging. If you get into a field and don’t feel like you belong, it’s hard to power through that. It makes it very unpleasant to be there. So I think that one of the ways social media can really help is by letting people see scientists who are not the stereotypical old white men. Talking about what being a scientist is really like, what the lifestyle is like, is really helpful for dismantling those stereotypes.
Your first book, The End of Everything, explored astrophysics but your next will popularise particle physics. Have you had to change your strategy when communicating different subjects?
This book is definitely a lot harder to write. The first one was very big and dramatic: the universe is ending! In this one, I’m really trying to get deeper into how fundamental physics works, which is a more challenging story to tell. The way I’m framing it is through “how to build a universe”. It’s about how fundamental physics connects with the structure of reality, both in terms of what we experience in our daily lives, but also the structure of the universe, and how physicists are working to understand that. I also want to highlight some of the scientists who are doing that work.
So yes, it’s much harder to find a catchy hook, but I think the subject matter and topics are things that people are curious about and have a hunger to understand. There really is a desire amongst the public to understand what the point of studying particle physics is.
Is high-energy physics succeeding when it comes to communicating with the public?
I think that there are some aspects where high-energy physics does a fantastic job. When the Higgs boson was discovered in 2012, it was all over the news and everybody was talking about it. Even though it’s a really tough concept to explain, a lot of people got some inkling of its importance.
A lot of science communication in high-energy physics relies on big discoveries, however recently there have not been that many discoveries at the level of international news. There have been many interesting anomalies in recent years, however in terms of discoveries we had the Higgs and the neutrino mass in 1998, but I’m not sure that there are many others that would really grab your attention if you’re not already invested in physics.
Part of the challenge is just the phase of discovery that particle physics is in right now. We have a model, and we’re trying to find the edges of validity of that model. We see some anomalies and then we fix them, and some might stick around. We have some ideas and theories but they might not pan out. That’s kind of the story we’re working with right now, whereas if you’re looking at astronomy, we had gravitational waves and dark energy. We get new telescopes with beautiful pictures all the time, so it’s easier to communicate and get people excited than it is in particle physics, where we’re constantly refining the model and learning new things. It’s a fantastically exciting time, but there have been no big paradigm shifts recently.
How can you keep people engaged in a subject where big discoveries aren’t constantly being made?
I think it’s hard. There are a few ways to go about it. You can talk about the really massive journey we’re on: this hugely consequential and difficult challenge we’re facing in high-energy physics. It’s a huge task of massive global effort, so you can help people feel involved in the quest to go beyond the Standard Model of particle physics.
You need to acknowledge it’s going to be a long journey before we make any big discoveries. There’s much work to be done, and we’re learning lots of amazing things along the way. We’re getting much higher precision. The process of discovery is also hugely consequential outside of high-energy physics: there are so many technological spin-offs that tie into other fields, like cosmology. Discoveries are being made between particle and cosmological physics that are really exciting.
Every little milestone is an achievement to be celebrated
We don’t know what the end of the story looks like. There aren’t a lot of big signposts along the way where we can say “we’ve made so much progress, we’re halfway there!” Highlighting the purpose of discovery, the little exciting things that we accomplish along the way such as new experimental achievements, and the people who are involved and what they’re excited about – this is how we can get around this communication challenge.
Every little milestone is an achievement to be celebrated. CERN is the biggest laboratory in the world. It’s one of humanity’s crowning achievements in terms of technology and international collaboration – I don’t think that’s an exaggeration. CERN and the International Space Station. Those two labs are examples of where a bunch of different countries, which may or may not get along, collaborate to achieve something that they can’t do alone. Seeing how everyone works together on these projects is really inspiring. If more people were able to get a glimpse of the excitement and enthusiasm around these experiments, it would make a big difference.